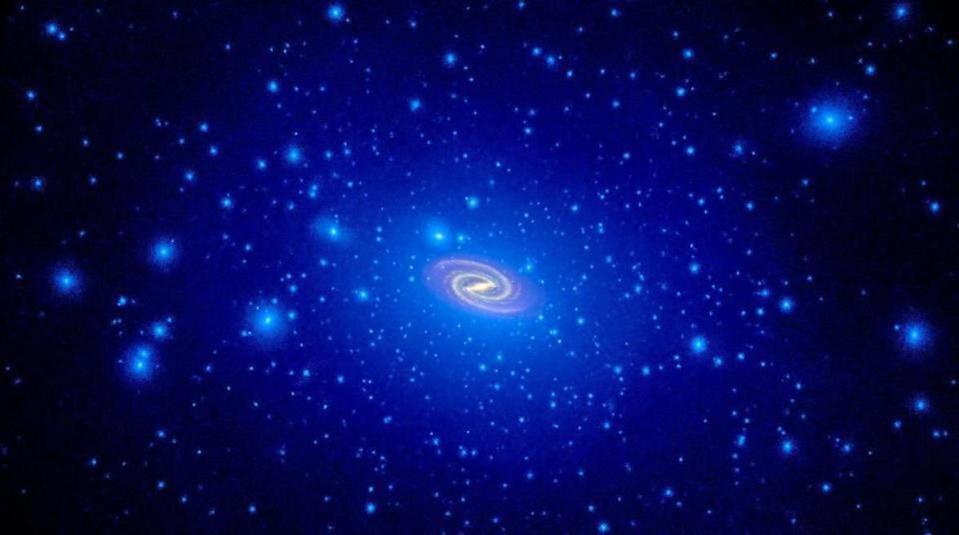
It’s hard to believe, but the idea that the Universe was dominated not by normal matter but rather by dark matter — a novel form of non-interacting matter that’s completely distinct from protons, neutrons, and electrons — goes all the way back to 1933. For decades, the overwhelming majority of the leading astronomers and physicists dismissed the idea as being ill-motivated, and it gained very little traction on both the theoretical and observational fronts throughout the ‘30s, ‘40s, ‘50s and ‘60s. It was only with the novel results and improved instrumentation initially leveraged by Vera Rubin and Kent Ford, and then further developed by Rubin on her own, that dark matter was brought into the cosmological mainstream in the 1970s.
But did either Fritz Zwicky, who first presented that 1933 evidence and even coined the term dunkle materie, which directly translates to dark matter, or Vera Rubin actually discover dark matter, or the overwhelming evidence in favor of it? Or is it unfair to say that dark matter was actually discovered by either of them, including up through and including the present day?
Although the astronomical evidence is overwhelming for dark matter’s existence, attributing dark matter’s “discovery” to any one individual misses the entire point of science, including how it’s conducted and how conclusions are reached. Here’s a context-rich history of dark matter that might surprise you in many ways.
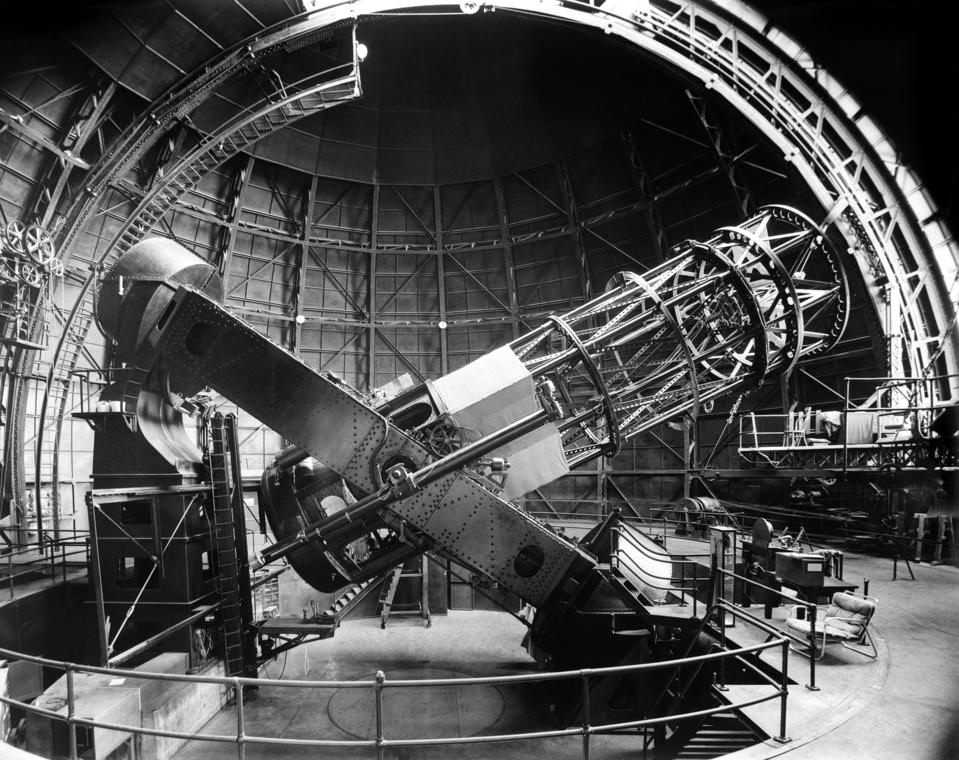
By the time the 1930s came along, even though that was some ~90 years ago already, astronomy was actually quite advanced as a science. Telescope apertures had already reached 100 inches (2.54 meters, which is larger than the Hubble Space Telescope’s mirror) and a 200-inch (5.1 meter) telescope was already under construction. We had learned that the spiral and elliptical nebulae in the sky were actually galaxies unto themselves, with their own stars and matter inside, located millions of light-years beyond the Milky Way. We knew the properties of stars and how brightness, mass, color/temperature, and ionization were all related. And we knew that the Universe was expanding, with light from more distant galaxies appearing systematically redshifted directly dependent on their distance from us. We had even measured the expansion rate: the first determination of the Hubble constant.
It was with this picture of the cosmos that the first hints of dark matter were discovered. In 1933, Fritz Zwicky was studying the galaxies in the Coma Cluster: a cluster of galaxies located just ~300 million light-years away. With over 1,000 identified galaxies in it today, it’s larger, richer, and more regular than the nearby Virgo Cluster (itself only ~50-60 million light-years away), and many of its galaxies are large, bright, and luminous.
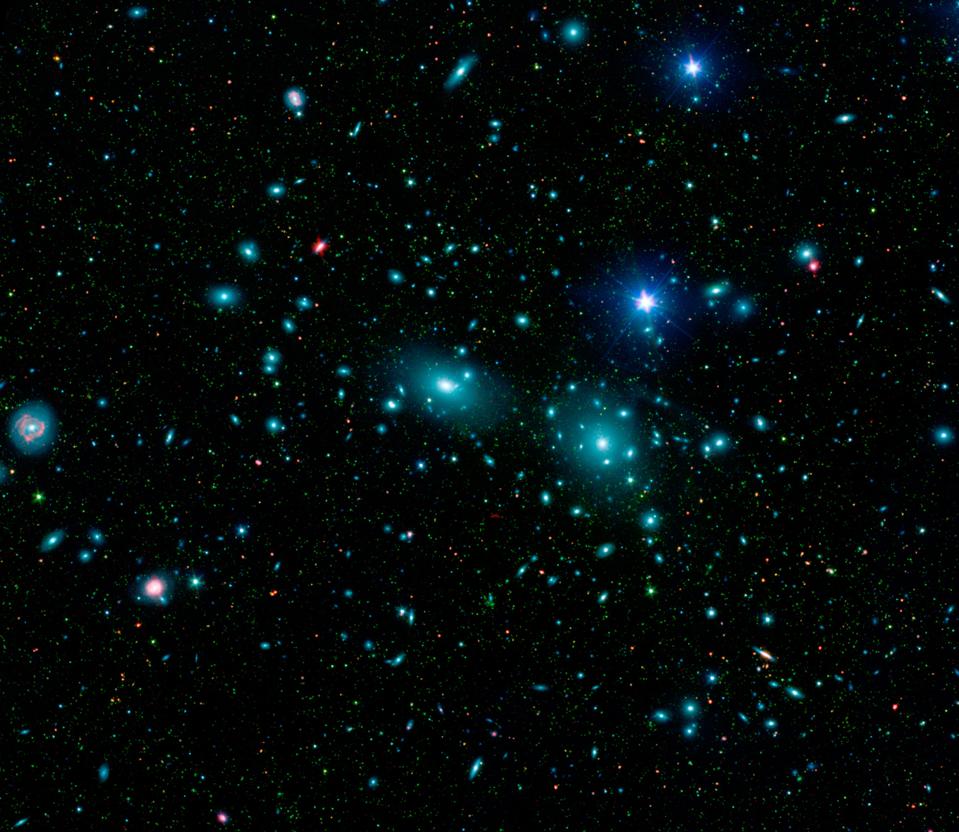
Even with the instruments available to Zwicky at the time, he was able to identify dozens of individual member galaxies of the Coma Cluster, including a number of bright spirals (mostly towards the cluster’s outskirts) and giant ellipticals (mostly towards the cluster’s center). When he measured the average redshift of the galaxies in the cluster, he got a value that corresponded to a speed of just about 2% the speed of light: the cluster was definitely speeding away from us along with the expansion of the Universe.
But Zwicky didn’t have to settle for the average value of the redshift across many different galaxies; he was able to measure the redshift of each member galaxy that he could resolve individually. Some of them — maybe even most of them — were moving with either the average value or a value close to the average, as indicated by their redshift. But others possessed redshift values that were much higher or much lower than the average, indicating that these galaxies making up the cluster were zipping around incredibly fast inside.
For that to be a stable configuration, there must be an enormous amount of mass holding this galaxy cluster together. Since there was no evidence that this cluster (or any similar cluster) of galaxies was flying apart, that mass must be present, even if we couldn’t see it.
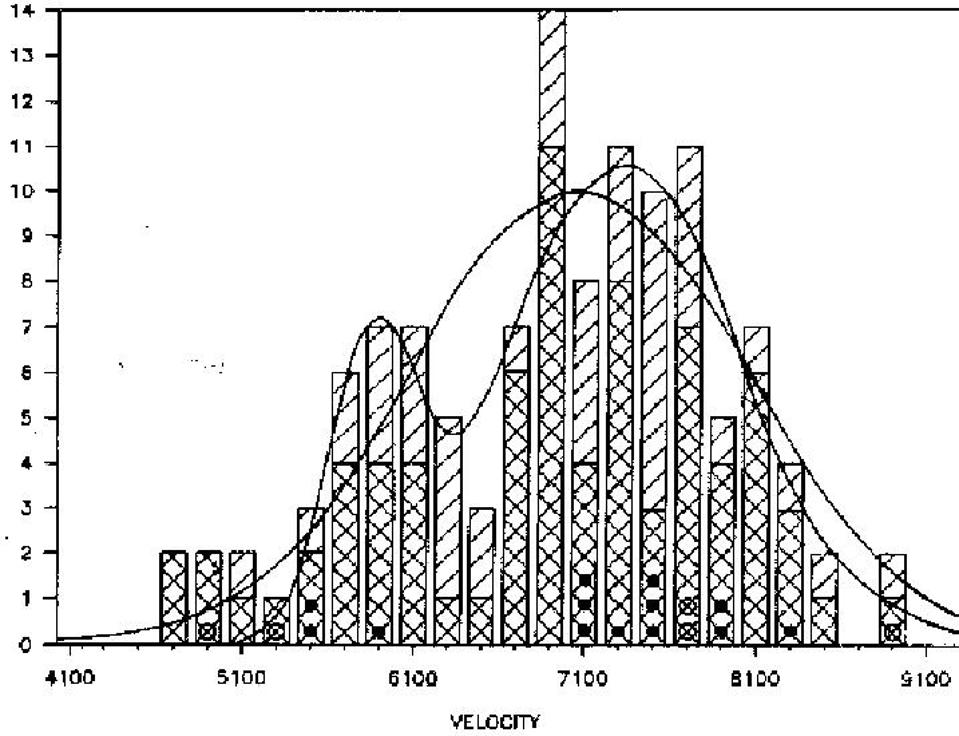
Zwicky’s reasoning was as follows:
- as astronomers, we know how stars work,
- and if we measure the starlight from all the galaxies in the cluster that we see, we can determine how much mass is in these galaxies and in the whole cluster,
- we also know how gravity and the expanding Universe works,
- so if we measure the average redshift of the cluster, we know how far away it is,
- and based on how fast we see these galaxies moving, there must be at least a certain amount of mass in there due to gravity.
When he compared the “mass from starlight” number with the “mass from gravitation” number, he realized that the latter number was over 400 times larger than the first number. Even if there were a small, unidentified error somewhere, he argued, this incredible mismatch meant, as a necessity, that there must be much more matter out there than the normal matter that we knew of could account for. He called this unseen matter dunkle materie: dark matter.
Zwicky was quite a gifted astronomer, but his conclusions were doubted by most professionals in the field, and for a multitude of good reasons. It wasn’t dogma, but rather the large cosmic unknowns that had yet to be resolved, that prevented the idea of dark matter from taking hold among the community.
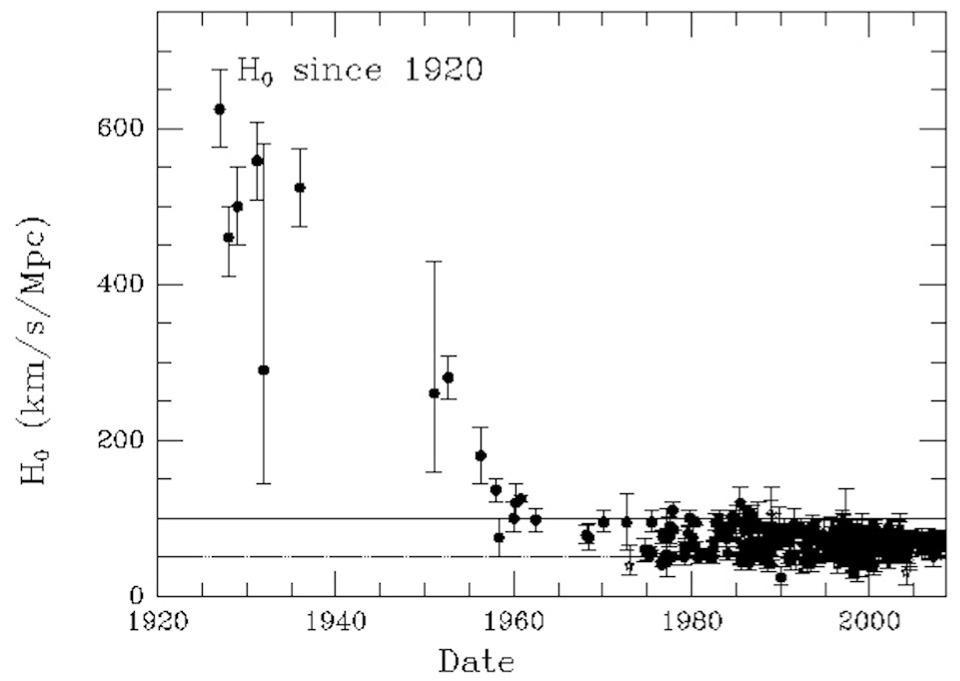
Here were some of the problems with Zwicky’s conclusions.
- Inferring the distance to the Coma Cluster: what you measure for a distant galaxy is just a redshift and an observed brightness. If you want to know the distance and don’t have a direct measurement (which we didn’t for any of Zwicky’s galaxies), you have to infer it from the Hubble constant, which was so absurdly high at the time that taking its value seriously implied a ~2 billion year old Universe: a Universe less than half the age of the Earth!
- Stars are not like the Sun, on average: after measuring the cumulative light from the stars in the observed Coma Cluster galaxies, Zwicky then assumed they had the same overall mass-to-light ratio that the Sun possesses. However, the light from galaxies is dominated not by stars like our Sun, but by hotter, bluer, more massive stars. Based on the observed light that Zwicky saw, there should have actually been multiple times the amount of mass inside that he assumed; the mass-to-light ratio is about three times the figure he used.
- There could be lots of normal, non-luminous matter present: this was perhaps the biggest objection to Zwicky’s conclusion. Why invoke some new type of matter to explain these galaxy’s motions within a cluster when the matter we know could be responsible? As long as it exists in any non-luminous form — gas, dust, black holes, plasma, etc. — then it need not even be present in the individual galaxies itself, but could be found between them. With such a massive unknown, why leap to the extraordinary conclusion that some new type of matter not only exists, but dominates the Universe?
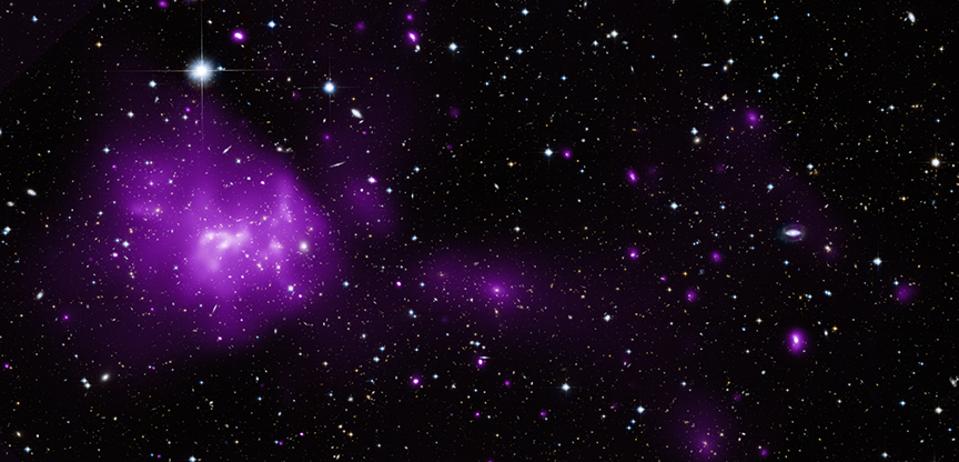
As the evidence continued to pour in through the decades, it became clear that these common objections to Zwicky’s conclusions were, in fact, quite legitimate. Walter Baade’s work demonstrated that the Hubble constant Zwicky was using was far too large (drastically changing the distance estimate of these galaxies), based on an error which failed to recognize that the Cepheid variables he was using to measure galactic distances came in fundamentally two different types. As our understanding of stars improved, we realized that they accounted for significantly more mass than previously anticipated. And, starting in the 1960s, we began measuring X-rays from galaxies within galaxy clusters and — later — from the intracluster medium itself.
Clearly, Zwicky’s mismatch of “a factor of ~400+” between the observed amount of matter present and the gravitationally inferred amount of matter necessary to hold galaxies clusters together was not correct. Estimates of the ratio of those two values dropped from ~400+ to ~160 to ~50 to less than a factor of ~10, with many assuming that all the hitherto undiscovered sources of normal matter would remove the need for “dark matter” almost everywhere. (The modern mismatch remains, but is only a factor of approximately 6.) But if you considered all of the astronomical data available, there were still some hints of dark matter’s existence that just wouldn’t go away.
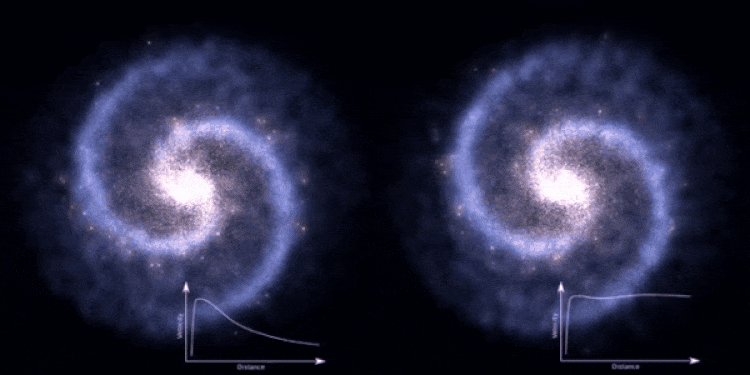
If starlight were a good tracer of matter — i.e., the matter is denser and more ubiquitous where the light from stars appears — then you’d expect the stars-and-gas in the interior regions of galaxies to zip around at greater speeds than the stars-and-gas on the outskirts. The assumption we initially made was that there was no such thing as dark matter, and that mass appears where light also appears: a totally reasonable one. But as our astronomical capabilities improved, bolstered by the development of multi-wavelength astronomy (including X-ray, radio, and infrared astronomy) and the ability to achieve higher resolution in measuring both different parts of the same galaxy and smaller differences in velocity from place-to-place, the Universe started telling a different story than what we had assumed.
Objects that were seen in the radio, first assumed and later confirmed to be galaxies, showed that the velocities of gas moving closest to the center was no larger than the velocities that could be measured farther away. More advanced measurements of galaxies in clusters showed a less-pronounced mismatch between the inferred mass from light and from gravitation than Zwicky first derived, but it was still present. And from the balance between gravitational potential energy and the speed of stars in small structures — star clusters, globular clusters, and dwarf galaxies — it became clear that some sort of unseen mass was required to explain these smaller galaxies as well.
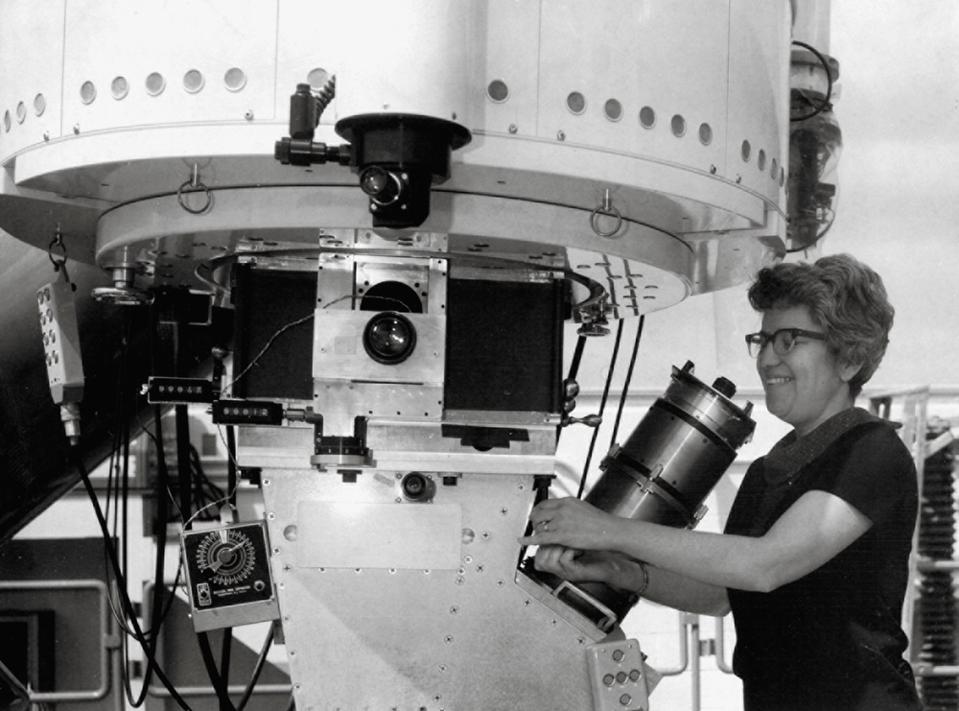
All of that sets the scene for the minefield that Vera Rubin walked into when she first began publishing her career-defining work on the rotation properties of individual galaxies throughout the 1970s. At this point in time, most astronomers were aware of Zwicky’s work, as well as the enormous sources of uncertainty surrounding the abundance of non-luminous matter that was still made from protons, neutrons, and electrons. A few galaxies exhibited rotation curves that were puzzling, and X-ray observations hinted at copious amounts of unseen but present “normal matter” within galaxy clusters. Importantly, the cosmological relationship between potential and kinetic energy in gravitationally bound objects — the virial theorem — had already become well-understood.
Working with her collaborator, Kent Ford, Rubin leveraged the new technology she had access to: Ford's intensified image tube cameras. The spectra that she was able to take of different parts of the same galaxy were able to achieve high spectral resolutions and to image faint parts of the galaxy — portions that were farther away from the center — than ever before. Beginning with the Andromeda galaxy and extending her work to about ten other spiral galaxies, she saw what no one else had seen before: that all spiral galaxies exhibited flat rotation curves, where the speed of the moving stars inside never dropped off to lower values, no matter how far away (within the observable limits) her measurements extended to.
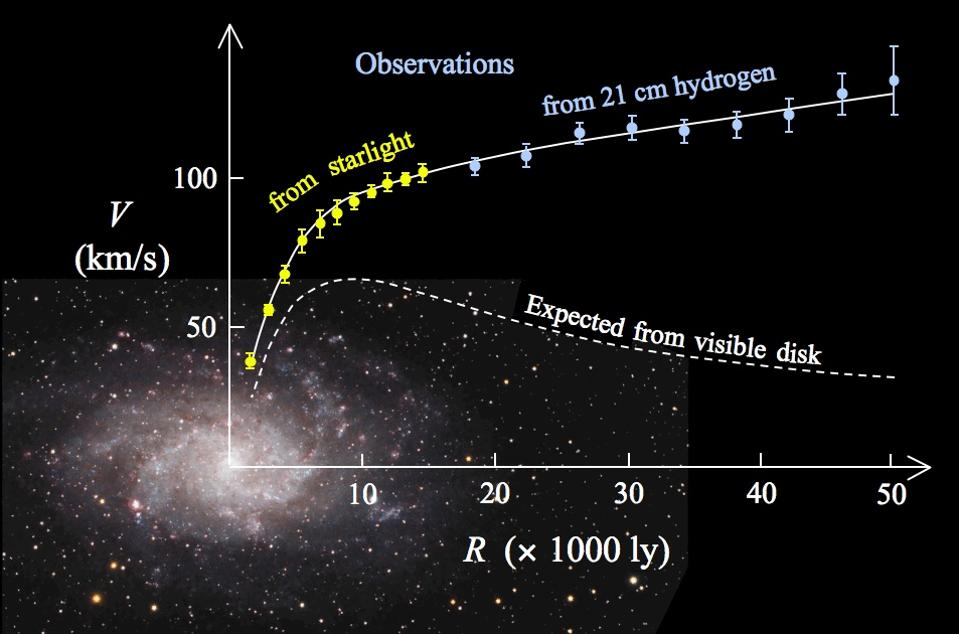
As groundbreaking as Rubin’s work was, it was equally controversial. Although the data was clear and unambiguous, the interpretation was not. The overwhelming majority of professionals in most sub-fields of astronomy were resistant to adding an entirely new form of matter to an already controversial Universe. Rubin was an observer, and the majority of referees — for non-scientific reasons ranging from inertia to clear-cut cases of sexism — demanded that she not include any interpretation of what the data meant. Nonetheless, Rubin stood her ground, continued presenting her results, and let the community make what they would of it.
By the late 1970s, most astronomers began to be convinced by the strength of not only her data, but of the improving data in other areas — from X-rays, radio, big bang nucleosynthesis, and the rapidly developing field of large-scale structure — that all pointed towards the existence of dark matter. Over the next few years, many observations, including:
- of elliptical galaxies at various distances from the center,
- of individual stars within dwarf galaxies,
- of speeds of galaxies as they fell into larger-scale structures,
- and the quantitative discovery of (insufficiently large) amounts of normal matter in the intergalactic medium,
all helped astronomers discover that the addition of a fundamentally new type of matter, what we today call dark matter, was required to explain all of the observations together.
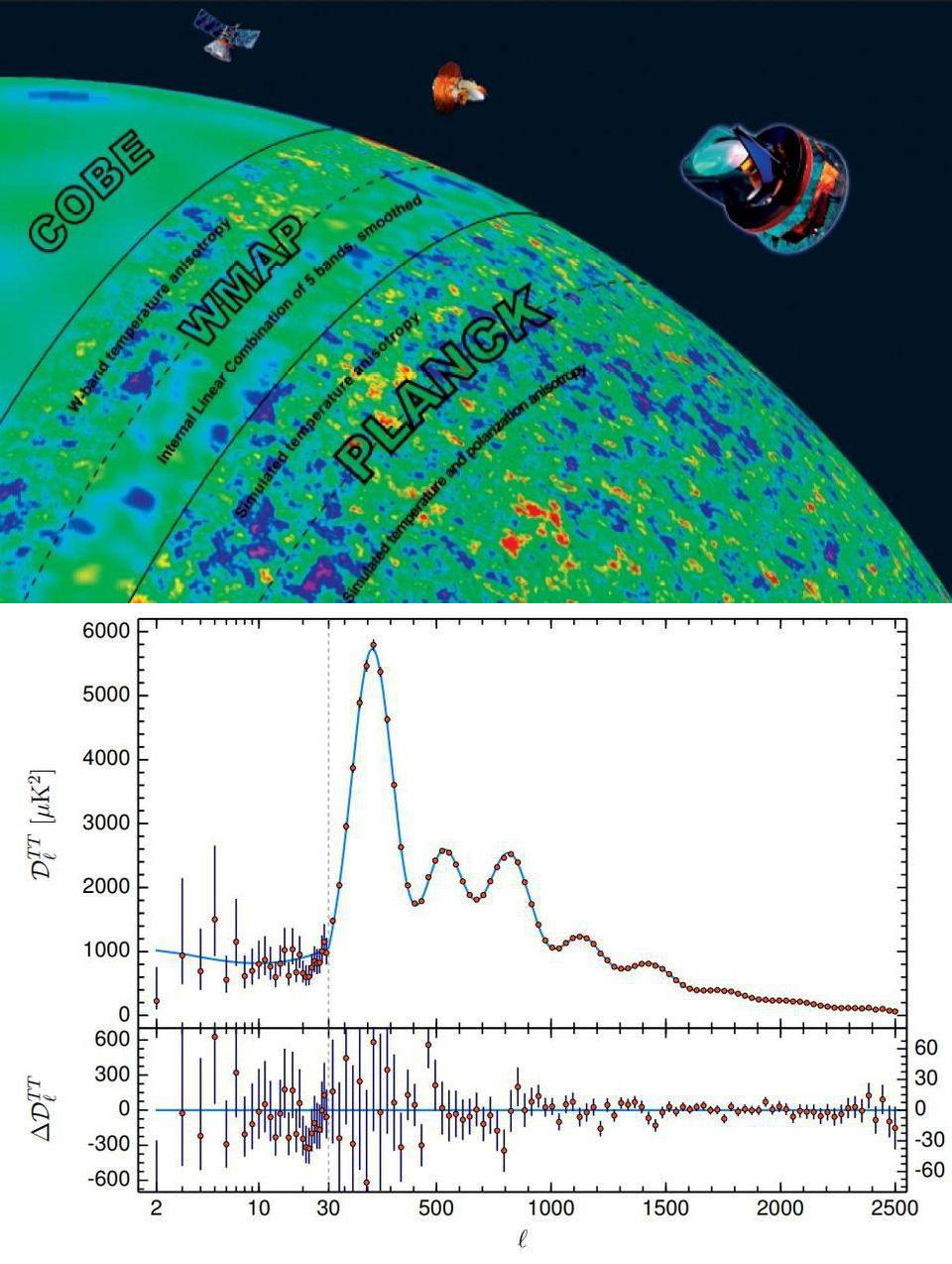
Today, the amount and quality of data at the disposal of all astronomers has improved by a factor of many thousands over what was available when Vera Rubin was engaging in her pioneering work. As is often the case, however, it’s unfair to credit a single person — even a single Nobel-worthy person who was snubbed as egregiously as Rubin was — with the discovery of dark matter. Rubin, although a vitally important part of the story in bringing credibility and evidence that simply could not be ignored to the astronomical community, didn’t perform her work in a vacuum.
She benefitted greatly from the instruments available to her and from the previous work done in the field. Zwicky’s work in the 1930s, Horace Babcock’s early measurements of Andromeda’s rotation, Jean Einasto’s improvements to our understanding of the virial theorem and its applications to cosmology, Ivan King’s work on star clusters and dwarf galaxies, and Jim Peebles’s Nobel-winning work on the large-scale structure of the Universe all influenced not only her, but the greater astronomical community.
In truth, dark matter has no unique, singular discoverer, but rather only became accepted because of the full suite of astronomical evidence. As improved data came in over the subsequent decades, the case for dark matter became overwhelming, so much so that the only viable alternatives must also invoke an additional field whose properties are indistinguishable from the effects of dark matter. It wasn’t Zwicky nor Rubin who discovered dark matter, but it was both of them who paved the way for our modern, superior understanding of what it is that truly makes up the Universe.