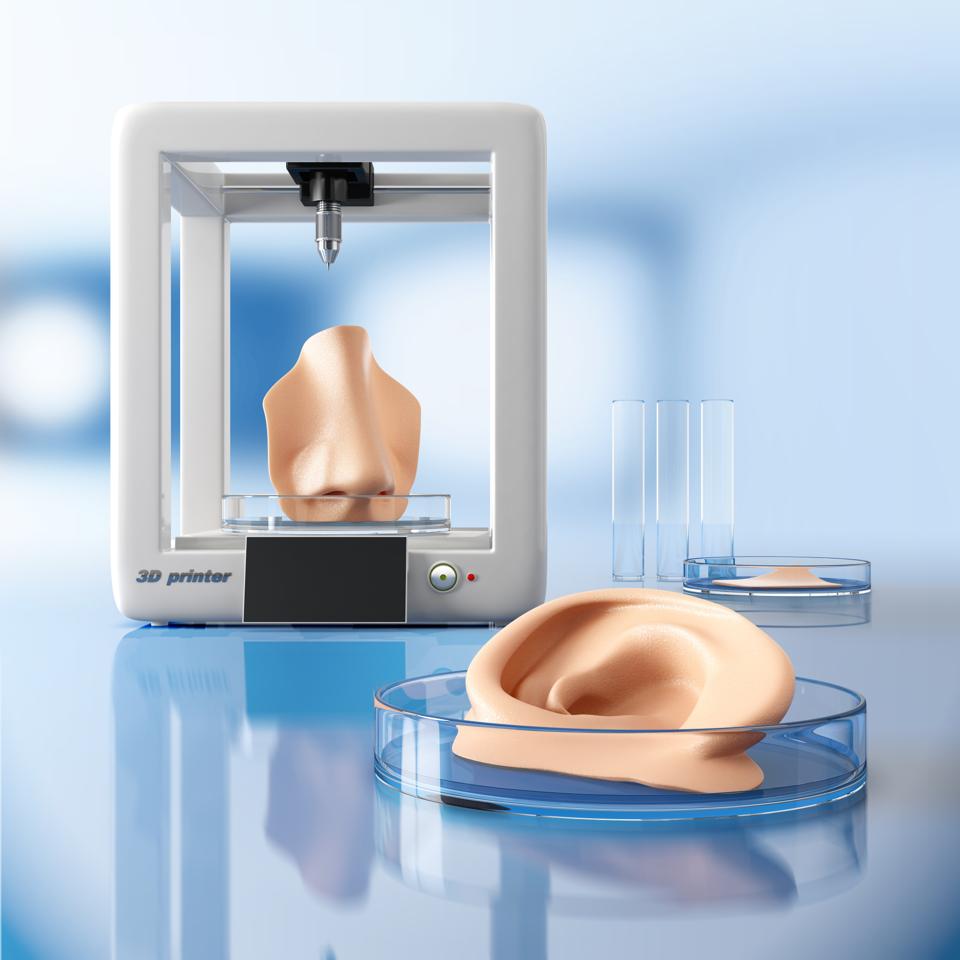
This story is part 11 of an occasional series on the current progression in Regenerative Medicine. In 1999, I defined regenerative medicine as the collection of interventions that restore to normal function tissues and organs that have been damaged by disease, injured by trauma, or worn by time. I include a full spectrum of chemical, gene, and protein-based medicines, cell-based therapies, and biomechanical interventions that achieve that goal.
Wounds come in many shapes and sizes. Some are small and heal quickly, causing few problems. Others are larger and slower to heal. Deep wounds that take especially long to go through the normal healing process, called chronic wounds, are of particular concern; these have a tendency to reopen and are often accompanied by infection and, eventually, scarring. Add to this the fact that chronic wounds are difficult to treat with currently available therapies, and you end up with a serious healthcare challenge.
Researchers from the University of Birmingham and the University of Huddersfield made use of an up-and-coming tissue engineering technique to develop a new treatment method for chronic wounds. In a paper published in APL Bioengineering, Richard Moakes et al. describe the process by which they managed to 3D print a functional skin equivalent that can be inserted into wounds to speed up recovery.
Our skin is made up of three layers: the epidermis, the dermis, and the hypodermis (Figure 1). The epidermis is the outermost layer and acts like a kind of armor, protecting our bodies from harm. Underneath the epidermis, we find the dermis. This is split into two parts, a thin upper layer called the papillary dermis, and the reticular dermis, a thick lower level; there is no clear demarcation between these two layers, meaning they overlap and intersect. Finally, all the way at the bottom, there’s the hypodermis. This layer is made up of thick connective tissue and adipose fat tissue.
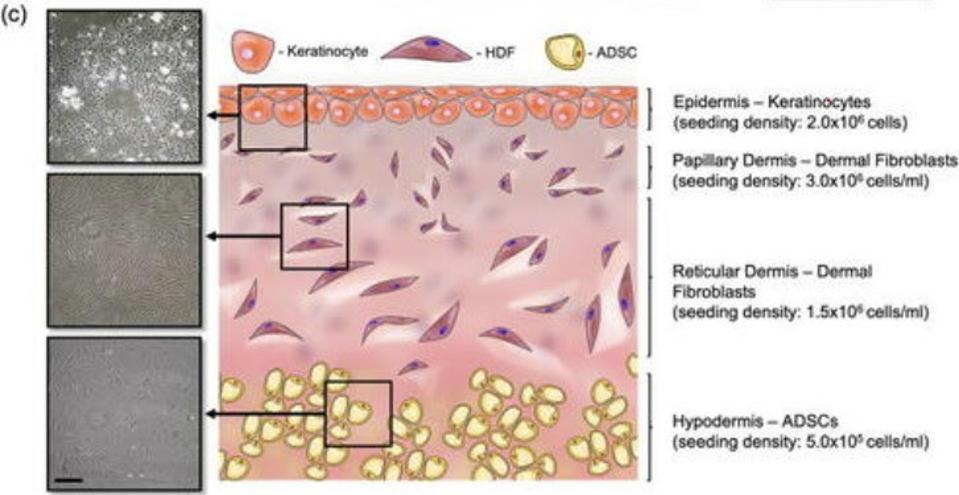
The complex, three-part nature of our skin is the main reason it’s so difficult to create a functional skin equivalent, with most attempts failing to accurately capture the subtle differences and gradients between each layer.
Moakes et al. mention that previous treatments have generally fallen along one of two approaches: either a biological approach, or a materials science approach. Both have seen significant advances in recent years. Even so, they still fall short.
The materials science path has yielded analogues with mechanical likeness — meaning they are structurally similar and “behave” in similar ways to real skin— but fail to provide the biological signals needed for rapid wound closure and regeneration of the damaged tissue.
The biological approach, in turn, has focused on using cells as the building blocks for the constructs. This results in preserved biological likeness, but structural inaccuracy; the cell-based models tend to be orders of magnitude too thin to support their intended functions. Attempts have been made to circumvent this issue through the use of “scaffolds.” These are three-dimensional structures that organize and hold the cells in ways that more closely simulate actual skin. Nevertheless, the scaffolds are by and large too uniform to fully recreate the mechanical complexity found across the three layers of our skin.
There is a third approach that has been gaining traction over the past few years: suspended layer additive manufacturing (SLAM). SLAM relies heavily on 3D printing, the process of creating 3D objects by laying down many thin layers of a material on top of one another.
Just as your average office computer requires ink, so, too, do 3D printers. In the case of 3D printing, the “ink” is the material —whether that be plastic, metal, etc.— of which the final structure will be made. When printing tissue analogues, researchers create a “bioink” made up of the cell types they’re trying to mimic. In this case, Moakes et al. used pectin and collagen, adjusting the ratio of the mix for each layer of skin.
Most bioinks need to be highly viscous to support themselves during the curing process. This also means they need to cure very quickly, often in a matter of seconds. Both factors create limitations that have previously restricted the complexity of the tissue equivalents researches could produce.
SLAM overcomes this issue by suspending the bioink in a fluid gel reservoir (Figure 2). Fluid gels are easily deformed, meaning the print head can move through them without difficulty, but are quick to bounce back to their original place, allowing the bioink to be trapped in its intended position without leaking. Fluid gels enable the bioink to be supported by something other than itself, meaning it can be of a lower viscosity and take more time to cure. The lower viscosity of the bioink permits the different layers to seep into one another, removing the need for rigidly pre-defined layers and, instead, creating a natural gradient. All in all this allows for much more complex designs and structures.
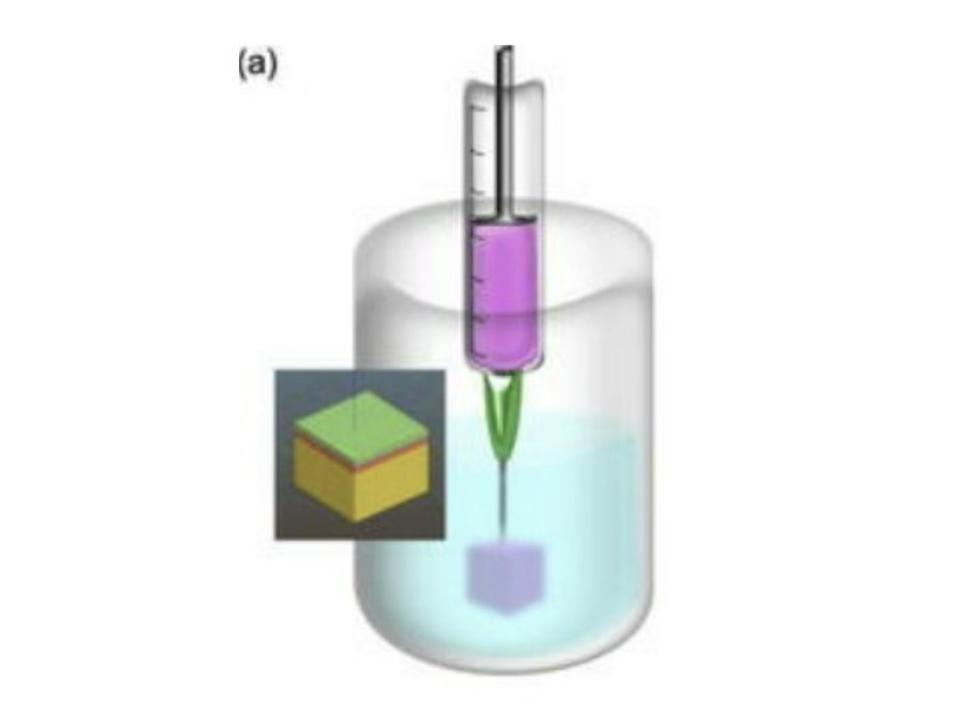
After using SLAM to produce a tri-layered skin equivalent, the researchers tested its usefulness as an implant for the treatment of wounds. They simulated a wound by cutting a hole in pig tissue. Although simulated wounds don’t necessarily mirror chronic wounds, Moakes et al. used it as a chance to test the integration and architectural deformation of the implant. It performed extremely well on both fronts.
The skin construct displayed signs of integration as early as 7 days post-implantation (Figure 3), even at the hypodermal level. This is especially note-worthy since recent studies have shown wound healing to happen bottom-up: from the deepest layer up towards the surface.
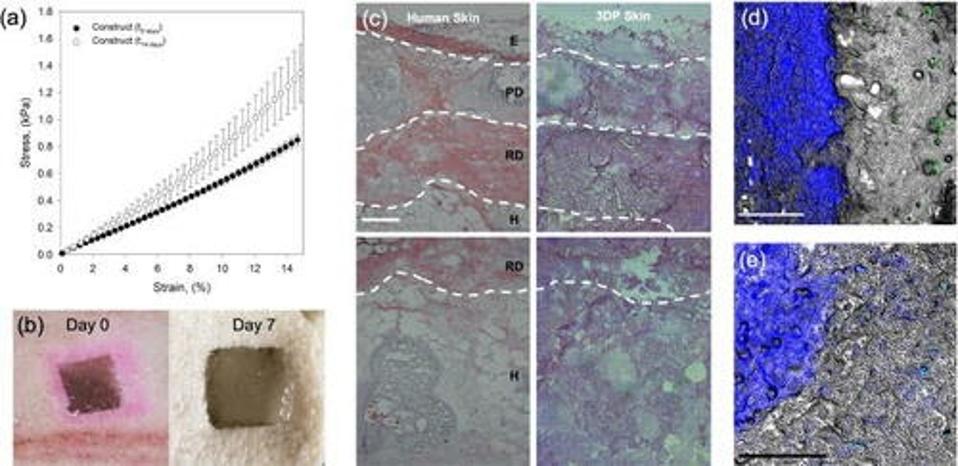
Not only does this study prove to be an exciting step towards the development of a new treatment method for chronic wounds, but it also offers the possibility of a truly bespoke approach. After all, each skin implant could be tailored to the wound in question, ensuring the best possible fit and the quickest possible healing.