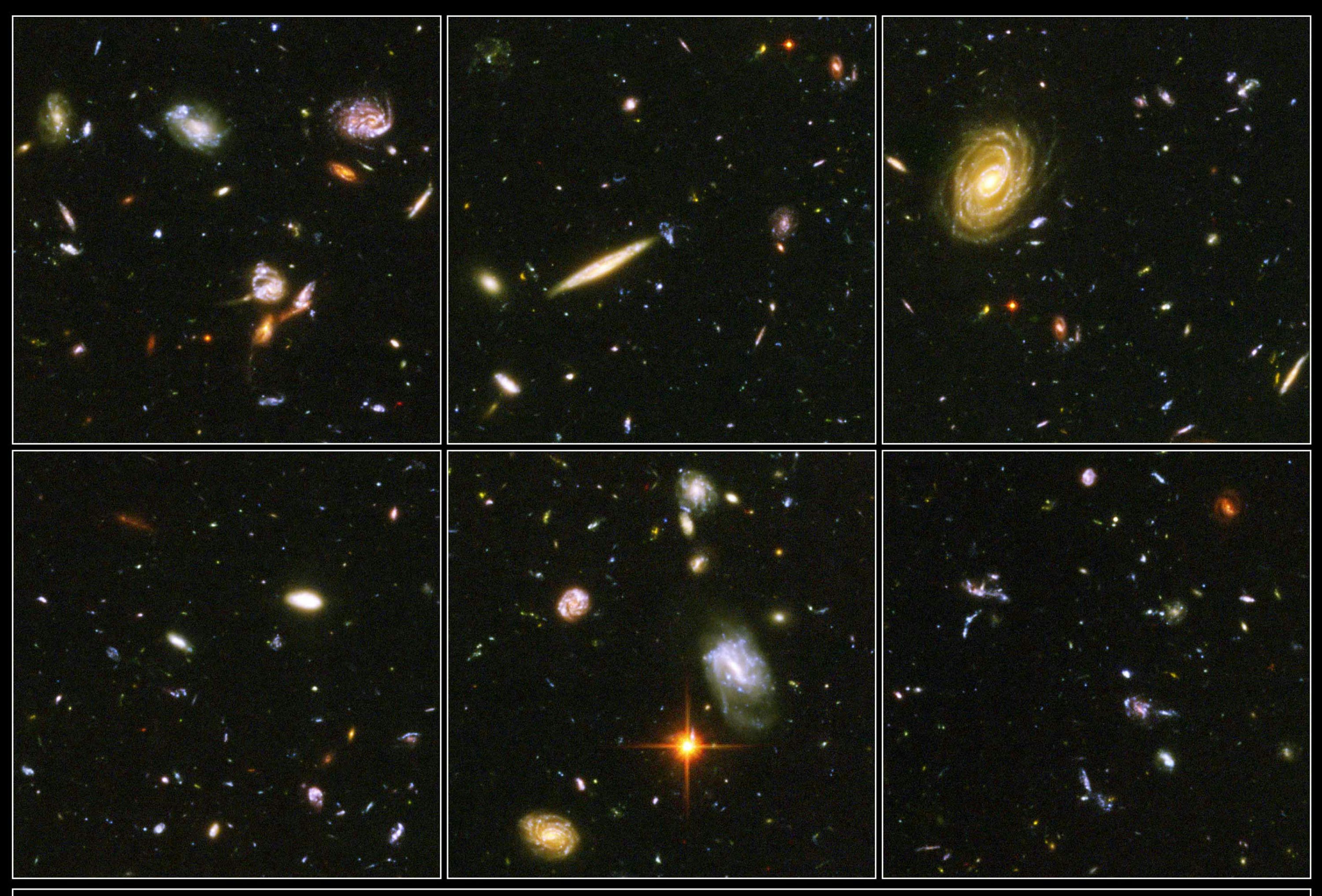
When astronomers stare into the deep universe or at the spectra of a distant star subtly hiding an exoplanet, they need to account for its movement. That relies on two phenomena — redshift and blueshift.
As objects move away from us, their light gets shifted into longer wavelengths or the red end of the spectrum — that’s redshift. Blueshift is the opposite, when light is shifted to shorter wavelengths on the blue side of the spectrum as an object comes towards us. These give essential clues on things like distance — and when staring at a distant galaxy, it lets you know how close you are to staring back at the dawn of time. This is important for telescopes like the James Webb Space Telescope, which its stakeholders have tasked with learning about the earliest galaxies in the infant universe.
To learn more about redshift and blueshift, Inverse spoke with Salvatore Vitale, an assistant professor of physics at the Massachusetts Institute of Technology. Vitale does data analysis on gravitational waves (ripples in space-time) charted by the Laser Interferometer Gravitational-Wave Observatory (LIGO) following huge events like black hole mergers. LIGO physicists work with astrophysicists to chart the distance to the gravitational waves they measure using redshift and blueshift — relying on multiple paths to understand the merger of some of the most potent forces in the universe.
Understanding blueshift and redshift
While redshift and blueshift sound esoteric, Vitale said we experience it in daily life with the sirens on ambulances and police cars. Sound and light have waves associated with them, so the analogy works: “Sound has a different distance to travel when the car comes towards you, instead of away from you,” Vitale tells Inverse.
As the siren approaches, the sound increases in frequency, and as it recedes, the sound decreases in frequency. This is more popularly known as the Doppler effect, which is the apparent difference between the frequency of waves an observer experiences relative to the source of the waves. The difference in motion between the observer and the source of the waves creates this effect.
When viewing objects in visible light, he added, “The universe has been kind to us.” Atoms always have characteristic frequency rates, which means we can say (for example) exactly what frequency the hydrogen in stars should be in a laboratory. So if hydrogen observed in a star system has a lower frequency, the star system is moving away, and vice versa.
Redshift and the universe’s expansion
Usually, redshift is discussed when talking about the expansion of the universe. An event 13.8 billion years ago, dubbed the Big Bang, caused the rapid inflation and expansion of space-time. Astronomers still see the echoes of that Big Bang, as objects in the universe are all receding from one another, thus experiencing some degree of redshift.
Those objects that are furthest away have the highest redshift. We know the universe is accelerating thanks to measuring the redshift of a particular type of star explosion (supernovas), called Ias. Astronomers have nicknamed these types of supernovas “standard candles” as they have a consistent luminosity. Since we know these supernovas’ inherent brightness, we can then chart their brightness in association with distance.
The surprise came in 1998, when astronomers announced supernovas were receding much faster than expected. Prior to this, astronomers assumed the universe was expanding at a constant rate. This led to the realization that the universe is accelerating as it expands, based on two independent studies of supernovas involving the Hubble Space Telescope and many other observatories to make sure they were seeing things correctly.
Why the universe is accelerating as it goes is subject to debate, but the leading hypothesis is a theoretical force called “dark energy.” Astronomers called the energy “dark” because we cannot sense it with our conventional telescopic instruments that look at waveforms of light. But we can measure the energy’s effect, as we can see the universe’s expansion is accelerating.
While we can’t pinpoint why the acceleration is happening, the discovery of this phenomenon gave the discovery teams a Nobel Prize in 2011.
As the James Webb Space Telescope prepares for first light, astronomers are especially excited to use its infrared capabilities to look back at the early universe at high-redshift objects, ones that will have come from shortly after a period called the Epoch of Reionization — where the first galaxies gave the universe a transparent sheen.
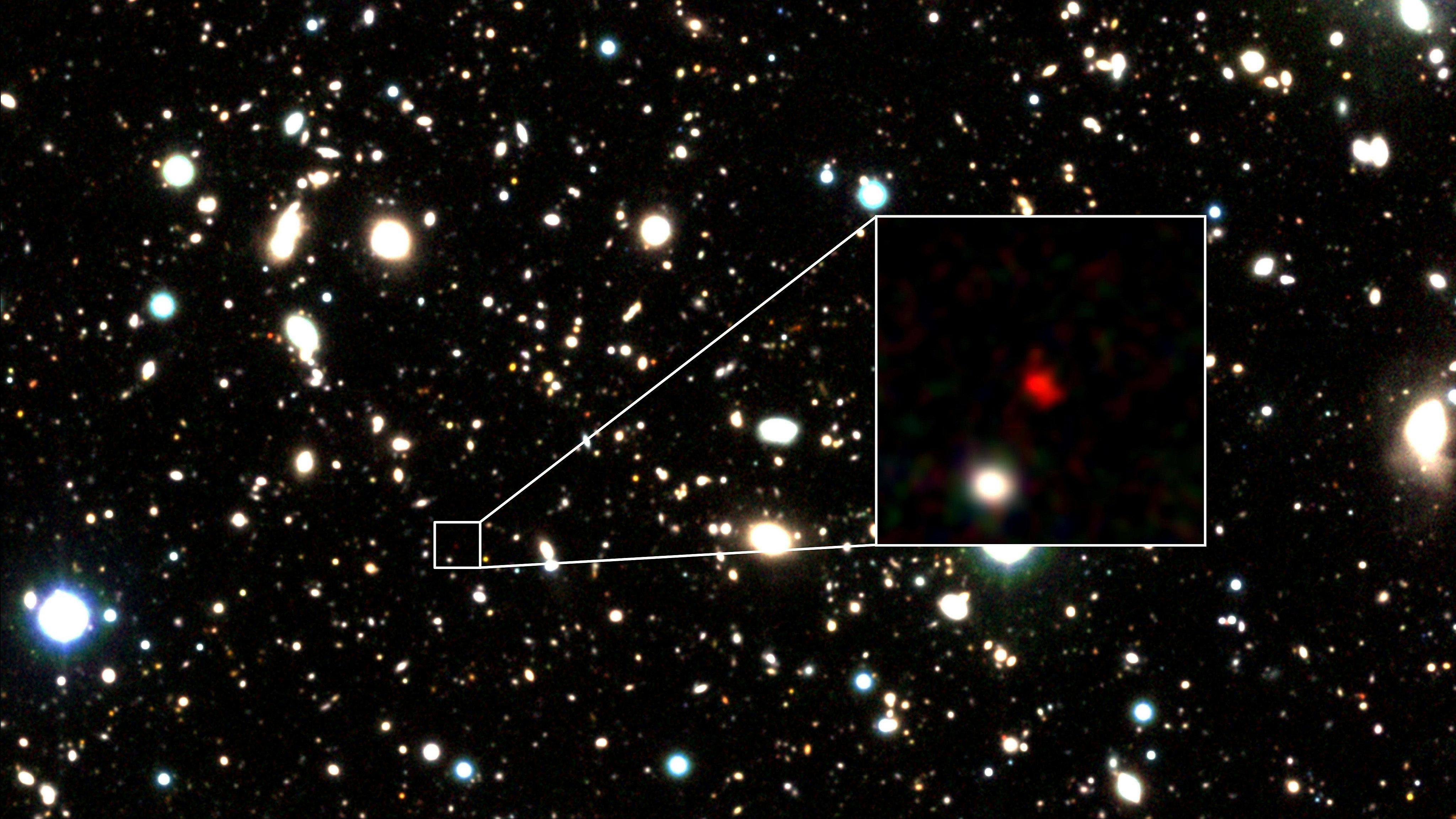
LIGO and redshift
LIGO is a planet-sized array of telescopes that chart huge events in space-time which also makes relies on redshift measurements. In October 2017, astronomers made the first confirmed detection of gravitational waves. They saw the effect of two crashing neutron stars — or the dense, city-sized remnants of stars left over after supernova explosions.
While the gravitational wave aspect of the initial discovery gets the most attention, what is also significant to astronomers is they charted this event in visible light waves, explains Vitale. For the same event, he said, “you can use the light from the source to get the redshift, and you can use the gravitational waves from the source to get the distance.” For this event and other gravitational waves, in other words, we need to use redshift to understand the distance.
How do we measure that distance? It dates back to Albert Einstein’s equations from 1916, when his theory of general relativity discussed how massive objects like these neutron star collisions could distort the fabric of space-time. Supercomputers in the 1990s finally allowed researchers to precisely chart these distortions. Astronomers were able to model the field equations Einstein predicted.
Today, it is common for gravitational wave scientists to model different types of mergers (such as between neutron stars, or between black holes) at different distances. Then when these events are seen in the real world, they have “waveforms” or model wavelengths they can use to match what they see with the predicted distance. That’s what happened successfully in 2017.
Distance and redshift together thus allow the astronomers to measure the universe's expansion, which is the deeper reason why that gravitational wave event was so meaningful that the teams received a Nobel Prize for their work.