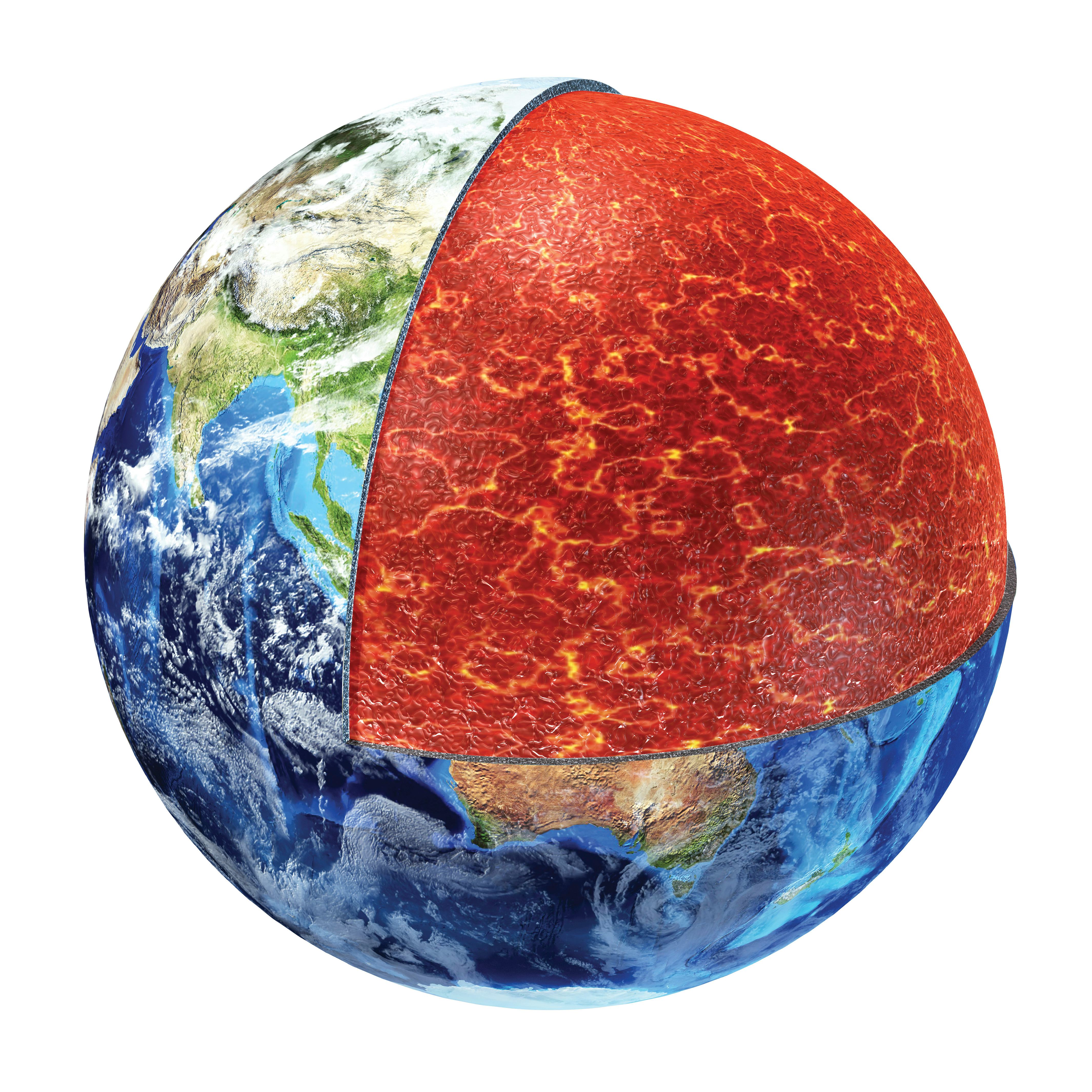
Dustin Trail began his academic career in computer science, but he quickly realized he had a more specific interest: the geology of the early Earth and the mysterious origins of life.
“We're at this exciting time in human exploration and searching for life on other planets and moons,” with Mars rovers and [the] James Webb Space Telescope,” Trail tells Inverse. “But we still do not know how or even when life started on our own planet.”
Today, Trail is a professor of Earth and Environmental Sciences at the University of Rochester. And together with Thomas McCollum, a scientist at the University of Boulder, published a new paper in the journal Science, characterizing for the first time the chemical properties of liquids circulating deep in the Earth’s crust more than four billion years ago. Those liquids — known as “lithospheric fluids” — may have carried minerals crucial to the development of early organic compounds up to warm water pools on the surface, setting the stage for early life to incubate.
A better understanding of how life was set up to evolve on Earth could not only solve a grand mystery about our own being, but it could also provide a more accurate template for searching for signs of existent or past life out in the universe. As Lunar and Planetary Institute Staff Scientist Laura Rodriguez notes in a commentary piece published alongside Trail’s paper in Science, zircons, pieces of the silicate mineral that were crucial to Trail and McCollum’s findings, are predicted to be common on Mars in rocks “altered by ancient fluids.”
What the researchers discovered about early Earth
Trail and McCollum studied zircons that formed in molten magma deep in Earth’s mantle around 4 billion years ago and which then migrated upward, crystallizing into the forms they possess today within wet hydrothermal vent systems. These are not, Trail notes, the cubic zirconia of the budget wedding rings, but “A zirconium silicate. So it’s got zirconium plus silicon plus four oxygens,” he says. “It's a remarkably durable mineral.”
Zircons are very hard, and so they persist despite being crushed, swept, and blown over the eons, but are also chemically durable: The zircons the two scientists studied for the paper retained a record of the chemical properties of liquid hydrothermal environments where they recrystallized, a sort of mineralogical time capsule.
What they discovered was that the lithosphere fluid where the zircons recrystallized was more oxidized than the mantle from which they came. For those needing a Chemistry 101 recap, oxidized environments contain more chemicals that donate electrons when interacting with other substances, with oxygen being the prime example. Rust is the result of oxygen interacting with metal and transferring electrons to create oxide compounds. A reduced environment is the opposite, containing more chemicals that like to receive electrons, such as hydrogen sulfide or ammonia.
Either way, Trail said, he had no idea what to expect to find at the outset of the study.
“This was the first study that deduced the oxidation state of a lithospheric fluid of that age, approaching 4 billion years,” he says. “If it had been really reduced, I would also have said it was unexpected.”
Still, as Rodriguez notes in her commentary, it is surprising to find oxidized fluids feeding into the sorts of surface pools that scientists hypothesize could have harbored the first forms of life since oxidized environments “are thought to not be conducive for origins-of-life chemistry,” she writes.
But what Trail and Mcollom were able to show was that this oxidized liquid would have absorbed metallic ions, atoms of iron, nickel, manganese, and calcium carrying positive electric charges, in this case, while flowing up to the surface. These are exactly the kinds of ions that could help along the types of prebiotic reactions scientists think would be necessary for the formation of life. They make that assumption, Trail says, based on how life today functions.
“All proteins require some metal — it’s called a cofactor, but it’s a kind of helper ion,” he says. “It basically needs it to function and do its job.”
Trail and McCollum first created synthetic zircons in a lab, crystallizing them in artificial environments with varying oxidative, temperature, and chemical conditions to establish a baseline against which to study and model the zircons from Western Australia.
After comparing the lab zircons to the ancient zircons, the two researchers were able to use modeling software to follow the chemical evolution of the lithospheric fluids from the mantle to the surface of the ancient Earth.
This is all possible because of the unique properties of the ancient zircons, Trail says.
Most of Earth’s early history is lost to geologists because the planet is so geologically active, most of the early crust has been recycled, subducted, and melted in the planet’s mantle, as well as chemically altered when on the surface and exposed to the elements.
”Because we have this active hydrologic cycle, it destroys rocks,” Trail says. “Once that happens, you have basically erased all previous information that rock could have held about its initial formation.”
Does this change our understanding of how life began on Earth?
Despite decades of research, no one knows just how life began on ancient Earth. But “it's reasonable to hypothesize that life could have started differently, or maybe even not at all if the chemical characteristics of the planet were different,” Trail says. So getting a better understanding of just what the chemical conditions were around the time life is believed to have got its start on Earth — somewhere between 4.5 and 3.5 billion years ago — is key to understanding the transition from prebiotic reactions to actual biology.
One idea suggests that some prebiotic chemistry pathways might require oxidized environments while others might need reduced environments, according to Trail. And some researchers have presented evidence, not yet conclusive, that the Earth’s early atmosphere was a reduced atmosphere, raising the interesting possibility that oxidized pools of liquid might have synergies with that atmosphere to accelerate the development of the chemistry that would make life possible.
“It's almost like mixing hot and cold water. Or salty and fresh water,” Trail says. “Those are two different chemical systems with unique properties.”
Now that there is data on the oxidative state of lithospheric fluids in the early Earth, laboratory scientists trying to simulate the probiotic soup of chemicals that might have led to the development of life will know how to calibrate the liquid components of their experiments.
And the results may help researchers looking for life off the planet as well. As Rodriguez notes in her commentary, the same calibration process used in this study could be used to study ancient Mars. Though since Mars is less active than Earth, Trail notes, Martian zircons might serve better as timekeepers than time capsules.
Zircons contain small amounts of two different isotopes of uranium, that is, forms of uranium with different numbers of neutrons in their atomic nucleus. Those two forms of uranium decay into two isotopes of lead, and since uranium decays at a steady rate, measuring the ratio of uranium to lead in a zircon provides an exact clock ticking down the time since that zircon crystallized.
“You basically get two clocks in one because there are two isotopes of uranium and two isotopes of lead. So you have a way to check your age,” Trail says. “I expect that zircons will anchor the chronology of many of the rocks that we find on Mars.”