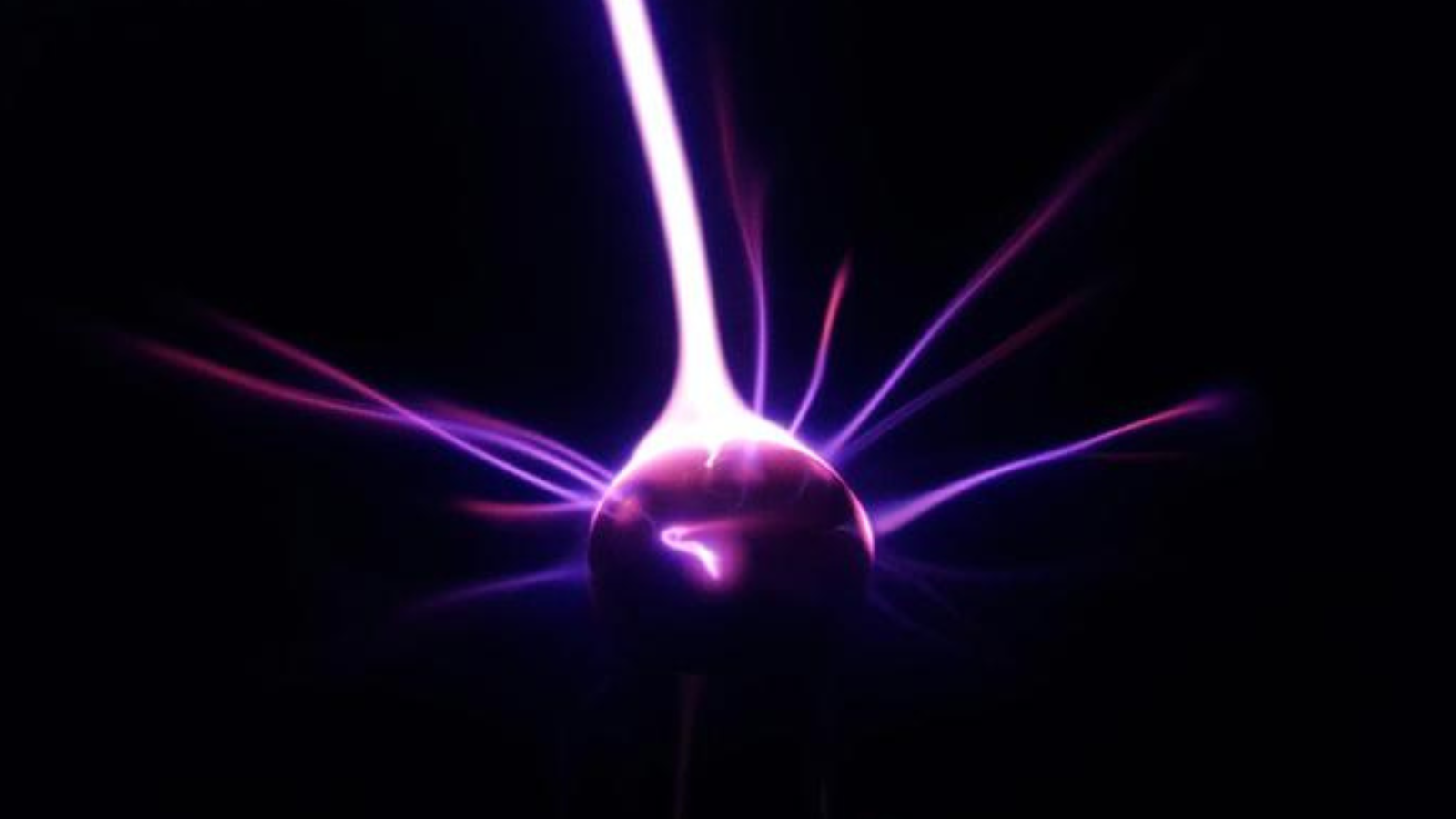
Scientists have determined a way to measure gravity on microscopic levels, perhaps bringing them closer to forming a theory of "quantum gravity" and to solving some major cosmic mysteries.
Quantum physics offers scientists the best description of the universe on tiny scales smaller than atoms. Albert Einstein's theory of general relativity, on the other hand, brings about the best description of physics on huge, cosmic scales. Yet, something is frustratingly missing even after 100 years of both theories passing a wealth of experimental verification.
As robust and accurate as the two theories developed at the turn of the 20th century have become, they have refuse to unite.
One of the primary reasons for this dilemma is that, while three of the universe's four fundamental forces — electromagnetism, the strong nuclear force and the weak nuclear force — have quantum descriptions, there is no quantum theory of the fourth: Gravity.
Now, however, an international team has made headway in addressing this imbalance by successfully detecting a weak gravitational pull on a tiny particle using a new technique. The researchers believe this could be the first tentative step on a path that leads to a theory of "quantum gravity."
"For a century, scientists have tried and failed to understand how gravity and quantum mechanics work together," Tim Fuchs, team member and a scientist at the University of Southampton, said in a statement. "By understanding quantum gravity, we could solve some of the mysteries of our universe — like how it began, what happens inside black holes, or uniting all forces into one big theory."
Related: 'Wavy space-time' may explain why gravity won't play by quantum rules
Gravity gets the 'spooky' treatment
It is maybe fitting that general relativity and quantum physics don't get along; after all, Einstein was never comfortable with quantum physics. This is because while quantum physics has many counterintuitive aspects, he found one in particular very troubling.
It was the notion of entanglement. At risk of simplification, entanglement has to do with coordinating particles in such a way that changing the properties of one particle instantly alters the properties of an entangled partner particle, even if the partner is located on the opposite side of the universe. Einstein called this "spooky action at a distance" as it challenged the concept of local realism.
Local realism is the idea that objects always have defined properties and that interactions between those objects are limited by distance and the speed of light, a universal speed limit introduced by Einstein as the foundation of special relativity. Special relativity is, in fact, the theory that led to the formulation of general relativity in the first place. Yet, despite Einstein's protestations, scientists have indeed proven that entanglement and other counterintuitive aspects of quantum physics are truly factors of reality at sub-atomic scales.
Such proof has been achieved with a multitude of pioneering experiments. Fuchs and colleagues, for instance, are following in the footsteps of physicists such as Alain Aspect, John Clauser and Anton Zeilinger, who won the 2022 Nobel Prize in Physics for experimentally verifying the non-local nature of entanglement.
In their new quantum experiment, the researchers, including scientists from Southampton University, Leiden University and the Institute for Photonics and Nanotechnologies, used superconducting magnetic "traps" to measure the weak gravitational pull on the smallest mass anyone has ever attempted to investigate in this way.
The tiny particle was levitated in the superconducting trap at temperatures of around -459.4 degrees Fahrenheit (-273 degrees Celsius), which is just a few hundredths of a degree above absolute zero, the hypothetical temperature at which all atomic movement would cease. This frigid temperature was needed to limit the vibrations of the particles to the very minimum. The team ultimately measured a gravitational pull of 30 "attoNewtons" on the particle.
AttoNewtons represent a measure of force; to give you an idea of how tiny the gravitational force on the studied particles was, one Newton is defined as the force needed to provide a mass of one kilogram with an acceleration of one meter per second per second. And 30 attoNewtons is equivalent to 0.00000000000000003 Newtons!
"Now we have successfully measured gravitational signals at the smallest mass ever recorded, it means we are one step closer to finally realizing how it works in tandem," Fuchs said. "From here, we will start scaling the source down using this technique until we reach the quantum world on both sides."
Team member and University of Southampton scientist Hendrik Ulbricht said this experiment paves the way for tests with even smaller masses, as well as the measurement of even smaller gravitational forces.
"We are pushing the boundaries of science that could lead to new discoveries about gravity and the quantum world. Our new technique that uses extremely cold temperatures and devices to isolate the vibration of the particle will likely prove the way forward for measuring quantum gravity," he concluded. "Unravelling these mysteries will help us unlock more secrets about the universe's very fabric, from the tiniest particles to the grandest cosmic structures."
The team's research was published on Friday (Feb. 23) in the journal Science Advances.