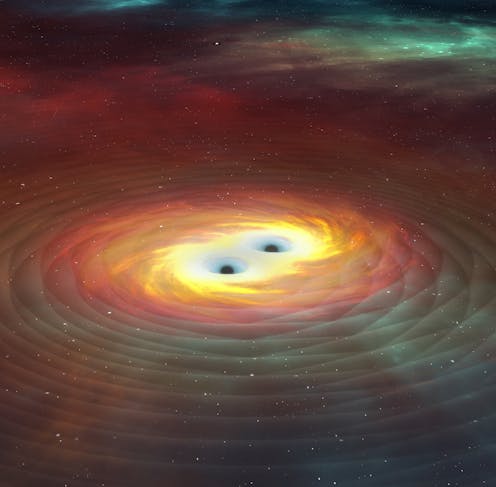
Galaxies, planets, black holes: to most people, everything about our Universe sounds and feels enormous. But while it’s true that much of what happens millions of light years away is large, there are also processes happening at the quantum end of the scale. That’s the branch of science which explains how nature works at very small scales – smaller than atoms. At this level, things behave in surprising ways.
Theoretical physicists Partha Nandi and Bibhas Ranjan Majhi explored the possibility that gravitational waves – ripples in space caused by massive objects moving or colliding – might exhibit quantum properties. They shared their findings with The Conversation Africa.
What are gravitational waves?
Simply put, they’re like tiny ripples in space, similar to the waves you see when you splash water. They occur when really heavy things in space, like stars or black holes, move around or crash into each other. These ripples then travel across space and carry energy.
They’re also far more than that: they are a method of communication. They carry information about massive cosmic events, helping scientists to “listen” to space in a way that wasn’t possible before their existence was confirmed.
In 1916 the legendary theoretical physicist Albert Einstein published a groundbreaking paper that laid out his theory of general relativity. He described gravity not as a force, but as the bending of space and time caused by massive objects. This bending affects how objects move, just like a heavy ball placed on a stretched rubber sheet makes smaller objects roll toward it.
Einstein accurately predicted the motion of planets, black holes, and even how light bends around massive objects – and the existence of gravitational waves rippling in space-time when those massive objects move or collide.
Read more: Curious Kids: what are gravitational waves?
It took nearly 100 years for Einstein’s hypothesis about gravitational waves to be confirmed. That’s when the Laser Interferometer Gravitational-Wave Observatory (LIGO) in the US detected these waves for the first time. It took such a long time because despite how huge they sound, gravitational waves are minute: they stretch or squeeze space by a factor 1,000 times smaller than the size of an atom. Special tools were needed to spot them and LIGO’s cutting-edge technology was up to the task.
You argue that some gravitational waves are quantum in nature. What does that mean?
“Quantum” is the branch of science that explains how nature works at very small scales – smaller than atoms. At this level, things behave in surprising ways.
For instance, tiny particles can behave like waves. They can also exist in more than one state at the same time, which is called superposition. Additionally, they can be mysteriously linked so that a change in one instantly affects the other, no matter how far apart they are. This is called entanglement.
Read more: Quantum entanglement: what it is, and why physicists want to harness it
Photons are a good example. These are particles of light, and scientists have proved that they behave in these “quantum” ways, such as being able to exist in superposition or becoming entangled with each other.
Entanglement is a kind of connection but it’s much deeper than a simple link. When two objects are entangled, they share something called a quantum state. This describes everything about a particle or system. It’s like a blueprint, but instead of fixed details, it gives the chance of finding the particle under different conditions, such as its position or speed.
When two objects share a quantum state, their behaviour becomes mysteriously linked. If you measure one object, the state of the other will immediately adjust to match, no matter how far apart they are. This is what makes entanglement so special and unlike anything we see in the everyday world.
What did your research reveal?
We hypothesised that gravitational waves could have both classical and quantum properties. The ones detected by LIGO so far follow classical behaviour, matching Einstein’s theory of general relativity.
But the current LIGO detectors aren’t sensitive enough to detect quantum effects, and there’s been no way to know whether our hypothesis is correct. So we modelled a detector similar to the latest generation of LIGO, which has mirrors attached to arms that can move and vibrate.
Classical gravitational waves cause the mirrors to move in specific ways, but in our study quantum gravitational waves – tiny ripples caused by particles called “gravitons” – affected the mirrors differently. They can make the mirrors’ oscillation modes become entangled: parts of the motion move together in ways that classical waves cannot create.
To visualise this, imagine two wind chimes far apart, swaying in sync because of an invisible breeze. Here, the quantum gravitational waves are like that breeze. They make distant objects move together in a way that classical gravitational waves cannot.
This suggests that at very small scales, gravitational waves may show quantum features, like entanglement, which can’t be explained classically. We’re not suggesting that all gravitational waves are quantum. However, this does not imply that all gravitational waves are quantum in nature. Instead, those originating from the early universe, approximately 13.8 billion years ago, may carry quantum signatures. These types of gravitational waves may encode information about the early universe, especially around the time of the Big Bang, and how they may have changed over time.
Why is this an important finding?
Confirming the quantum nature of gravitational waves bridges Einstein’s relativity with quantum mechanics, solving a puzzle that has challenged physics for decades: the difficulty of reconciling the principles of general relativity, which describes gravity on a large scale, with the laws of quantum mechanics, which govern the behaviour of particles at the smallest scales.
This breakthrough could revolutionise our understanding of the universe. The quantum nature of gravitational waves could help advanced sensors detect faint cosmic signals and provide insights into the universe’s origins, black hole behaviour, and the fabric of reality. While LIGO has already made great progress in measuring gravitational waves, exploring their quantum side opens up a new field of physics.
Read more: Gravitational waves: will the global south provide the next pulse of gravity research?
It’s important to note that more research will be needed to test and replicate our findings in different experimental settings. We’re far from the only people studying these phenomena and we hope our findings will strengthen the efforts of South African institutions such as the National Institute for Theoretical and Computational Sciences (NITheCS) and the Astrophysics Research Group at Stellenbosch University which contribute to gravitational wave astrophysics through data analysis, collaboration and theoretical work.
Advances in technology will also play a key role in expanding quantum gravitational wave research opportunities. The LIGO-India observatory, due to become operational by 2030, will be one such possible experimental setting.

Partha Nandi receives funding from the University of Stellenbosch. as a posdoctoral fellowship.
Bibhas Ranjan Majhi does not work for, consult, own shares in or receive funding from any company or organisation that would benefit from this article, and has disclosed no relevant affiliations beyond their academic appointment.
This article was originally published on The Conversation. Read the original article.