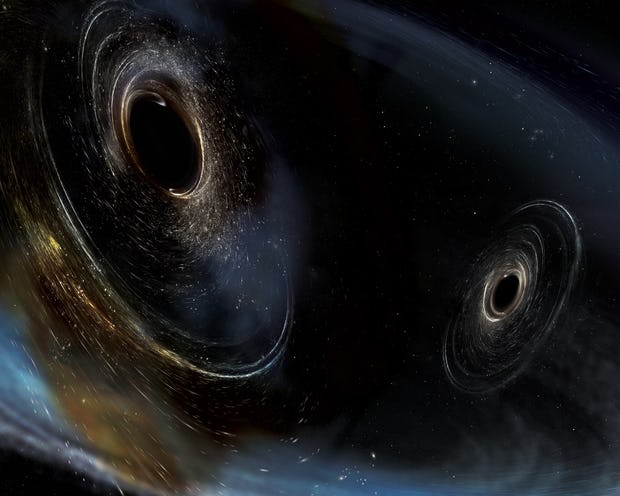
In the grand scheme of things, the structure of a black hole is pretty simple. All you need to know is its mass, electric charge, and rotation, and you know what the structure of space and time around the black hole must be. But if you have two black holes orbiting each other, then things get complicated.
Unlike a single black hole, for which there is an exact solution to Einstein’s equations, there is no exact solution for two black holes. It’s similar to the three-body problem in Newtonian gravity. But that doesn’t mean astronomers can’t figure things out, as a couple of recent studies show.
Although Einstein’s equations don’t have an exact solution for a binary black hole system, there are aspects of binary black holes that the equations predict. One of these is known as spin-orbit resonance. When a black hole rotates, the structure of space around it is twisted in the direction of rotation, known as frame-dragging.
When two black holes orbit each other closely, the frame-dragging of each black hole affects the rotation of the other. As a result, the two black holes will tend to enter a resonance, where the rotations either align in the same way (parallel) or opposite (anti-parallel). If spin-orbit resonance is real, then binary pairs should tend to have one of these orientations.
One recent study suggests this is true. In it, the team looked at gravitational-wave data from known black hole mergers and found that their rotations tend to be parallel or anti-parallel. Given the small sample size, and the fact that black hole binary rotations are never exactly aligned, there isn’t enough data to confirm the effect, but the data we have points in that direction.
One of the challenges to measuring black hole spin is that the signal is rather weak. The gravitational waves we measure from distant black hole mergers are so faint that it’s easy to get lost in the noise. Observatories such as LIGO and Virgo need to make extremely sensitive measurements, and their data must be filtered through computer models. Its the combination of data processing and computer simulation that makes the mergers detectable. Adding spin to the mix makes things even more difficult.
But in a second paper, the team looked at how we could get better results. They found that the signal for spin resonance is strongest when they are just about ready to merge. That makes sense since that’s when they are closest together and when frame-dragging is strongest. But currently, the rotation information for binary black holes is found by looking at gravitational waves while they are still orbiting each other. The team showed how models can analyze the near-merger signal instead, getting much better results. By applying this new method to black hole mergers, they should be able to confirm spin-orbit resonance in the near future.
Gravitational-wave astronomy is still a new field, and we’re still learning how to capture and analyze the data. As these new studies show, gravitational waves hold a great deal of information, and with a bit of digging there’s plenty more, we can uncover.
This article was originally published on Universe Today by Brian Koberlein. Read the original article here.