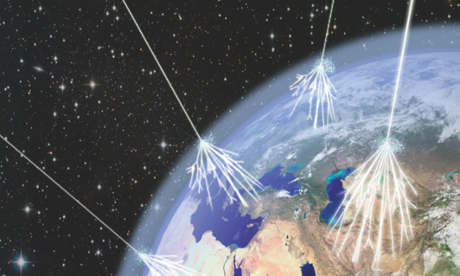
On Wednesday the whole of year six from my daughter’s primary school came to visit UCL. They saw the Slade School of Fine Art, the department of Earth Sciences and finally my department, Physics & Astronomy. That’s two classes of 30 children each, and for those of you not familiar with today’s jargon, ‘year six’ is ages 10 and 11. Or ‘fourth year juniors’, as we were called in my day.
The first thing I showed them was a cosmic ray detector. It was ticking away in on the bench at irregular intervals, about one tick per second, and an oscilloscope trace was registering each pulse.
“Can any of you guess what this is detecting? What is making those ticks?” I asked, expecting the answer no.
“Is it Moo-Ons?” said a boy standing by the machine. I was impressed. “How did you know that?”
“It says ‘Moo-On Detector’ on the box”
Never underestimate children, especially once they learn to read.
He did not, however, know what muons (normally pronounced mew-on) actually are. They are fundamental particles, very like electrons but 207 times more massive.
The muons being detected in the laboratory at UCL come from high-energy particles from space - called ‘cosmic rays’ - which continually bombard the upper atmosphere. When they hit the atoms in the atmosphere, they smash up the atomic nucleus and produce a spray of hadrons - particles containing quarks and gluons.
These hadrons hit more atoms and smash them up too, and a shower of particles is created. As the shower develops, the number of particles increases, but the average energy per particle decreases. At some point the energy becomes low enough that the new particles can’t smash up any more atoms, and the shower dies down.
The fact that it dies down is good for us, because it reduces the radiation dose we get at ground level. The maximum point of the shower, when it has the highest number of particles, takes place around 10,000m, which is unfortunately about the height at which aeroplanes cruise. Over a year’s work, airline crews can receive about twice the normal background radiation dose of someone working on the ground, and they have to monitor their exposure.
Muons are produced when some of the hadrons in these showers decay. In fact, muons were first discovered, in 1936, in measurements of cosmic rays. They are one of the few high-energy particles to reach the ground, because they are unique: they are massive enough that the electrons in atmospheric atoms don’t stop them, and (unlike hadrons) they don’t interact via the strong force, and so they rarely collide with the atomic nucleus.
Some of the cosmic rays which hit the atmosphere release energies far higher than those achieved at the highest energy particle colliders built on the Earth. The fact that this has been going on for billions of years is one of the reasons we could be confident that the current highest-energy machine, CERN’s Large Hadron Collider, would not, through some bizarre freak of unknown physics, cause a catastrophe.
Since these cosmic rays come for free, and the LHC does not, you might wonder why we didn’t just use the cosmic rays to hunt for the Higgs boson in the first place. After all, as I mentioned, that’s how we discovered the muon.
To answer that question, you need an estimate of the number of cosmic rays with sufficient energy that impact upon the Earth’s atmosphere. On a recent trip to CERN, a colleague pointed me at the Batchelor’s thesis of Josua Unger of the Technical University of Dresden, which addresses exactly that question. The estimate Unger comes up with, under various reasonable assumptions, that a Higgs boson is produced somewhere in the atmosphere about once every 8 seconds¹. For These Higgs bosons are produced mainly when gluons, inside the various produced hadrons, collide and fuse together. And they would be highly boosted - that is, travelling very, very fast.
That is not a very high Higgs rate, and the news gets worse, because to have a chance of seeing them, the bosons would have to decay to something distinctive - preferably muons. They do this sometimes, and we have measured Higgs bosons decaying to muons (via W or Z bosons) at the LHC, but the fraction of them that do so is tiny.
Of course, you would also have to cover the whole world with muon detectors something like the one in our lab at UCL, or probably better, because you would have to measure their energy and direction quite precisely to reconstruct the Higgs boson. Overall, it is quicker, simpler and cheaper to build a hadron collider.
Nevertheless, I find it strangely reassuring that nature is steadily making Higgs bosons, up there in the sky, without our help.
¹With a pretty large uncertainty that says there’s a 65% chance the value is between one every 6.5 seconds and one every 30 seconds.
Jon Butterworth’s book Smashing Physics is available as “Most Wanted Particle” in Canada & the US. He is also on Twitter.