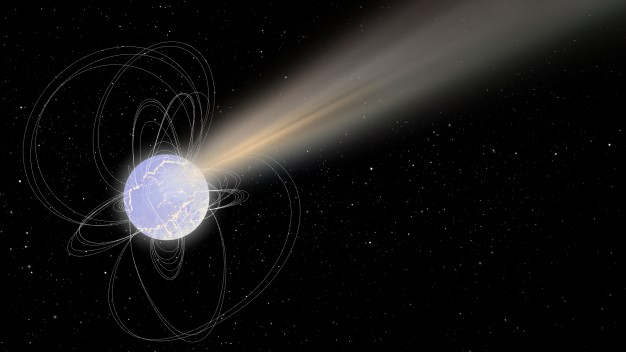
New research has revealed that different types of neutron stars, born when massive stars die, behave in a similar way. This might sound like a minor result, but in the grand scheme of things, it's a finding that further supports the idea that these extreme dead stars — so dense a tablespoon of one equals something like the weight of Mount Everest — could be behind mysterious blasts of radiation called Fast Radio Bursts (FRBs).
Millisecond-long bursts of radio emission FRBs seem to come from sources beyond the limits of the Milky Way, yet since their discovery in 2007, their origins have remained shrouded in mystery. There is one possible suspect, however: Highly magnetic neutron stars, or magnetars.
And the team behind the new discovery, including researchers from the Max Planck Institute for Radio Astronomy (MPIfR) and the University of Manchester, found that magnetars indeed share a relationship between the pulse structure and rotation that also present in other so-called "radio-loud" neutron stars.
The discovery of a similar "universal scaling" between different sorts of neutron stars hints at the plasma processes that may be responsible for these radio emissions themselves; it also leads scientists to interpret structures seen in FRBs as being the result of a corresponding rotational period, the team says.
"When we set out to compare magnetar emission with that of FRBs, we expected similarities," Michael Kramer, first author of a paper on the findings and the director of MPIfR, said in a statement. "What we didn’t expect is that all radio-loud neutron stars share this universal scaling.
Related: Supernova explosion’s weird leftovers may contain a super-dense star
Neutron Stars! Pulsars! Magnetars! Oh My!
Neutron stars form when massive stars run out of fuel for nuclear fusion. Once a star runs out of such fuel, the energy that's been supporting them against the inward, crushing force of their own gravity ceases to exist. This then causes the outer layers of the star to be blown away, resulting in massive supernova explosions while the core itself collapses.
That collapse continues until electrons and protons in the region smash together and create a sea of neutron-rich matter, which prevents the stellar core from crushing down ever more. Any more and the core would eventually create a black hole. Sans black hole, however, the result of the core-collapse process is a body around the width of a city here on Earth — about 12 miles (20 kilometers) — and matter that's incomprehensibly dense. Recall the Mount Everest example.
This isn’t the only extreme quality the gravitational collapse causes, however. Just as an ice skater can speed up their spin by drawing in their arms, the stellar cores that create neutron stars reduce in radius and therefore "spin up." This spin-up process can affect a core quite a bit. Some younger neutron stars, for instance, can rotate as fast as 700 times every single second. And if they are blasting out jets of radiation that sweeps over Earth, like a beam from a cosmic lighthouse, the fast-spinning neutron star is called a pulsar. Neutron stars that rotate hundreds of times per second are called millisecond pulsars.
Though pulsars are notable for their periodic nature, "Rotating Radio Transients," on the other hand, are neutron stars that emit more sporadic and less well-timed blasts of radio waves.
Additionally, when the magnetic field lines of stellar cores "crush in" during this collapse, magnetic fields that are a thousand billion times stronger than the magnetic field of the Earth are created. But some neutron stars have more powerful magnetic fields than even that, pushing limits 1,000 times stronger than that of a "normal" neutron star.
These are known as magnetars.
Astronomers know of around 30 magnetars to date, and around 6 of those have been seen to emit radio waves, leading scientists to theorize that FRBs come from magnetars outside the Milky Way — extragalactic magnetars.
Finding a connection between all 'radio loud' neutron stars
The team set about investigating the possible magnetar-FRB link by looking at individual pulses of the 6 known magnetars in detail. They then probed the sub-structure of the pulses with the Radio Telescope Effelsberg located in Bad Münstereifel, Germany, one of the largest fully directable radio telescopes on Earth.
To the team’s surprise, the fast-rotating millisecond pulsars and Rotating Radio Transients have similar pulse structures. This led them to a universal scaling relationship for magnetars and the other forms of neutron stars that connect pulse structure to the rotational period, whether these extreme stars are rotating every few milliseconds or in around 100 seconds.
"We expect magnetars to be powered by magnetic field energy, while the others are powered by their rotational energy," Kuo Liu, team member and a scientist at MPIfR, said in the statement. "Some are very old, some are very young, and yet all seem to follow this law."
In other words, the fact that all these radio-wave-blasting neutron stars behave like magnetars suggests that the intrinsic origin of the sub-pulse structure must be the same for any and all neutron stars emitting radio waves.
"If at least some FRBs originate from magnetars, the timescale of the substructure in the burst might then tell us the rotation period of the underlying magnetar source," Ben Sappers, team member and a researcher at the Jodrell Bank Center for Astrophysics, said in the statement. "If we find this periodicity in the data, this would be a milestone in explaining this type of FRB as radio sources."
The team’s research was published on Nov. 23 in the journal Nature Astronomy.