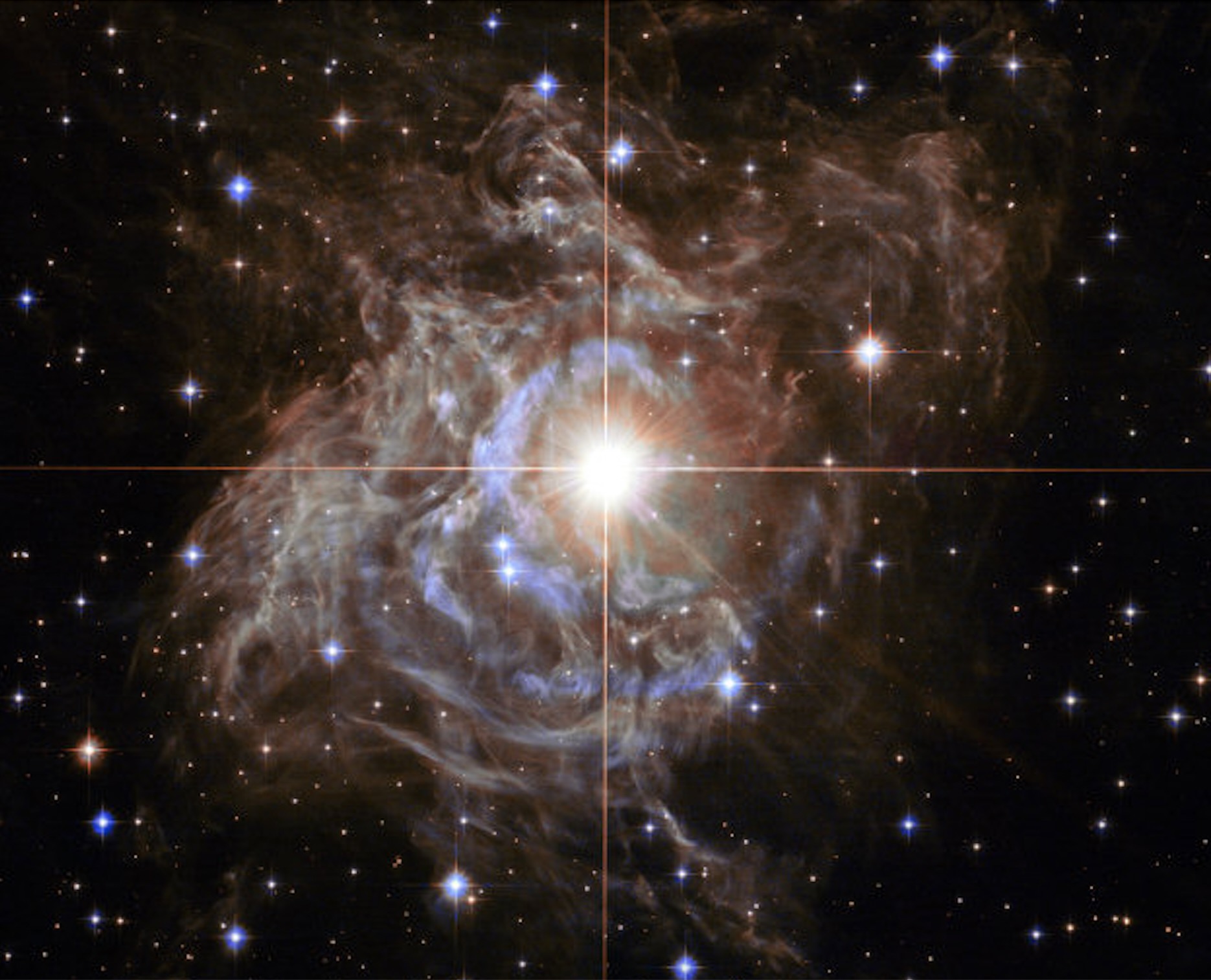
The most accurate observation to date of distant stars that periodically change in brightness may spark a rethink of the rate at which the universe expands — perhaps by settling a longstanding problem in cosmology, or deepening it.
The observation confirms a disparity that exists between the two major methods of measuring how fast the universe is expanding, conforming with one but not the other, a new study reports.
Researchers with the Stellar Standard Candles and Distances group used data collected by Europe's Gaia spacecraft to study Cepheid variable stars, which pulsate in a regular manner, providing a way of accurately measuring cosmic distances. The Cepheid star measurement technique expands on other methods, such as one that relies on observations of Type 1a supernovas.
Related: Hubble telescope refines universe expansion rate mystery
The light output of supernovas, mammoth explosions that occur at the end of big stars' lives, is so uniform they are referred to as "standard candles" and form an important part of what astronomers call the "cosmic distance ladder." The Cepheid star distance measurement method adds another "rung" to that metaphorical ladder, and this new research has strengthened that rung.
"We developed a method that searched for Cepheids belonging to star clusters made up of several hundreds of stars by testing whether stars are moving together through the Milky Way," study co-author Richard Anderson, a physicist at the École Polytechnique Fédérale de Lausanne (EPFL) in Switzerland, said in a statement.
"Thanks to this trick, we could take advantage of the best knowledge of Gaia's parallax measurements while benefiting from the gain in precision provided by the many cluster member stars," Anderson said. "This has allowed us to push the accuracy of Gaia parallaxes to their limit and provides the firmest basis on which the distance ladder can be rested."
The cosmic distance ladder is also used to measure the expansion rate of the universe, known as the Hubble constant. This new recalibration of the Cepheid "rung" deepens a problem with the rate at which the universe expands, which has come to be known as the "Hubble tension."
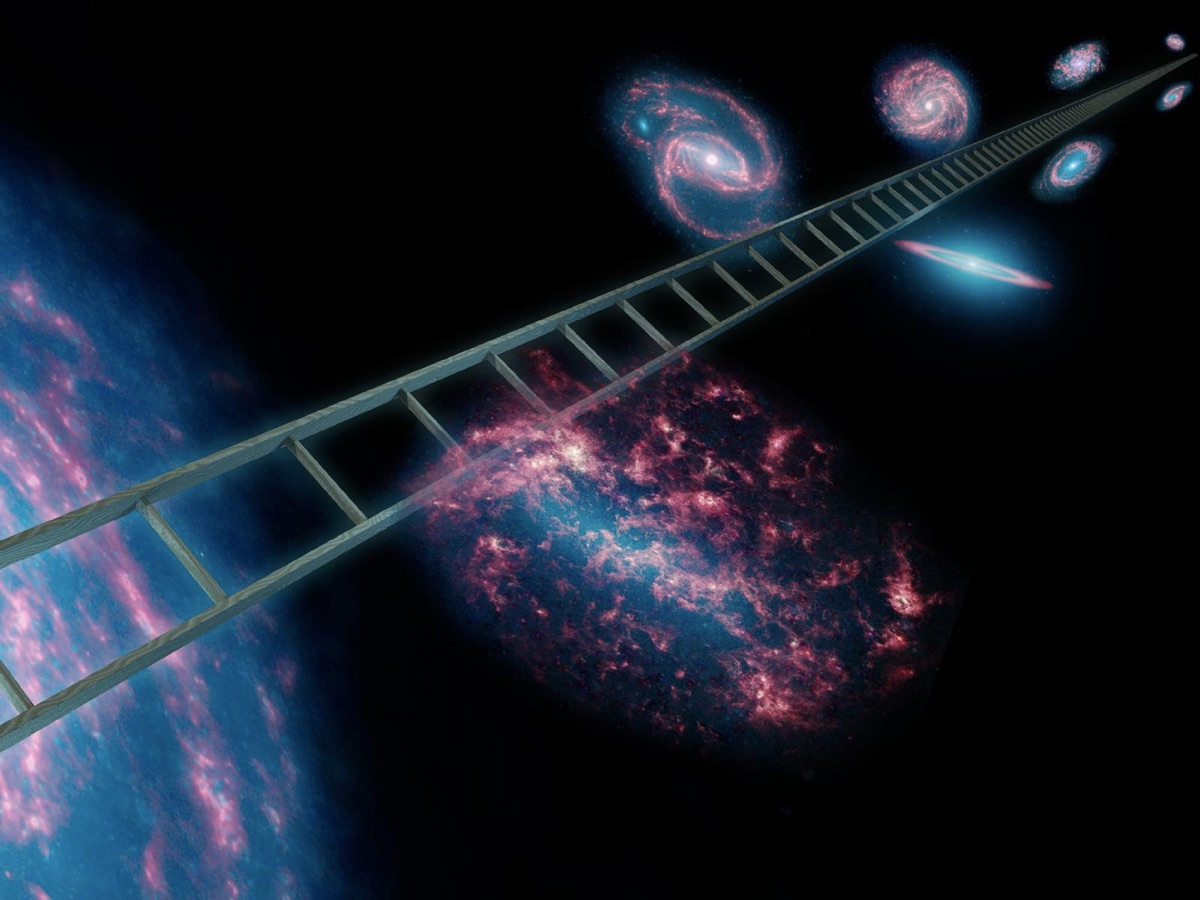
What is the Hubble tension?
In the early 20th century, shockwaves rippled through physics and astronomy when Edwin Hubble uncovered evidence that the universe is not static, as was believed at the time, but is actually expanding. This rate of expansion therefore became known as the Hubble constant.
This concept underwent a major shakeup in the late 1990s, when astronomers discovered via the observation of distant supernovas that, not only is the universe expanding, but it is doing so at an accelerating rate. Since then, measuring the Hubble constant has become a thorny issue for astronomers and cosmologists, because there are two major ways of determining this value — and they don't agree.
One method uses galaxies' velocities as a function of distance to deliver a Hubble constant value of about 73 ± 1 kilometers per second per megaparsec (km/s/Mpc), with 1 Megaparsec representing around 3.26 million light-years. This is known as the "late time" solution, because it comes from measurements of the universe in recent times.
The other method of measuring the Hubble constant looks at the light from an event shortly after the Big Bang called "the last scattering," in which electrons combined with protons to form the first atoms. As free electrons had previously scattered photons (particles of light) dramatically, preventing them from traveling very far, this event meant that light was suddenly allowed to travel through the cosmos freely.
This "first light" is now seen as the cosmic microwave background (CMB), and it almost uniformly fills the cosmos, barring tiny variations. When astronomers measure these tiny variations in this fossil radiation, it predicts a modern-day value for the Hubble constant of around 67.5 ± 0.5 km/s/Mpc.
The differences between the two estimations of the Hubble constant have strangely only grown as measuring techniques for both have been refined and have become more precise. This 5.6 km/s/Mpc difference, and the general trouble surrounding it, is referred to as the "Hubble tension." It's a serious issue for cosmologists, as it suggests there is something wrong with our understanding of the basic physical laws that govern the universe.
Related: The universe is expanding so fast we might need new physics to explain it
Cepheid variables pick a side
Anderson explained why a difference of just a few km/s/Mpc in the Hubble constant matters, even given the vast scale of the universe. (The width of the observable cosmos alone is estimated to be around 29,000 MPC.)
"This discrepancy has a huge significance," Anderson said. "Suppose you wanted to build a tunnel by digging into two opposite sides of a mountain. If you've understood the type of rock correctly and if your calculations are correct, then the two holes you're digging will meet in the center. But if they don't, that means you've made a mistake — either your calculations are wrong or you're wrong about the type of rock."
Anderson said that is analogous to the Hubble tension and what's going on with the Hubble constant.
"The more confirmation we get that our calculations are accurate, the more we can conclude that the discrepancy means our understanding of the universe is mistaken, that the universe isn't quite as we thought," he added.
The improved calibration of the Cepheid variable measurement tool means that this technique finally "takes a side" in the Hubble tension debate, providing agreement with the "late time" solution.
"Our study confirms the 73 km/s/Mpc expansion rate, but more importantly, it also provides the most precise, reliable calibrations of Cepheids as tools to measure distances to date," Anderson said. "It means we have to rethink the basic concepts that form the foundation of our overall understanding of physics."
The team's results have other implications as well. For example, the more accurate Cepheid calibration also helps to better reveal the shape of our galaxy, study team members said.
"Because our measurements are so precise, they give us insight into the geometry of the Milky Way," study lead author Mauricio Cruz Reyes, a Ph.D. student in Anderson's research group, said in the same statement. "The highly accurate calibration we developed will let us better determine the Milky Way's size and shape as a flat-disk galaxy and its distance from other galaxies, for example."
The new study was published last week in the journal Astronomy & Astrophysics.
Follow us on Twitter @Spacedotcom and on Facebook.