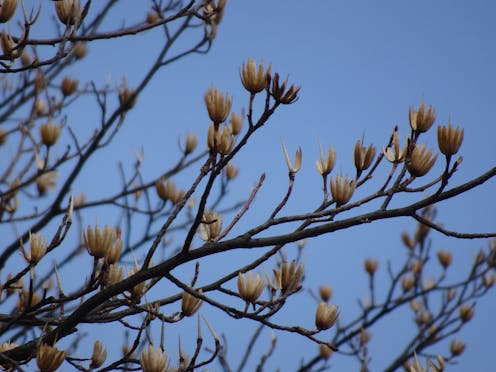
For as long as scientists have studied trees, we have categorised them into two types based on the sort of wood they make. Softwoods include pines and firs and generally grow faster than hardwoods, like oaks and maples, which can take several decades to mature and make a denser wood.
However, our recent research has uncovered something completely new: a third category we’re calling “midwood”. This discovery could prove to be valuable in the fight against rising carbon dioxide (CO₂) levels in Earth’s atmosphere – the primary cause of climate change.
Trees are natural carbon sinks. This means they absorb huge amounts of CO₂ from the air and store it in their wood. The tulip tree (Liriodendron tulipifera), also known as the yellow poplar, is a top performer in carbon capture. In the mid-Atlantic US, forests dominated by tulip trees store between two and six times more carbon than forests where other species prevail. The tulip tree is already popular in plantations in parts of south-east Asia and cited as a good choice for carbon capture for gardeners and urban planners in the US.
This species, along with its close relative the Chinese tulip tree (Liriodendron chinense), belongs to an ancient lineage dating back 50-30 million years — a period marked by significant shifts in atmospheric CO₂. Only these two species survive. And until recently, their chemistry and structure, which might tell us why these trees are so good at capturing carbon, were largely unknown.
Traditional methods for analysing the internal structure of wood overlook the differences between living and dried wood, the latter being much easier to study. That’s a problem because, without water, wood at the molecular level changes. The challenge is to observe wood that still retains its water.
We overcame this by using a technique known as low-temperature scanning electron microscopy in the Sainsbury Laboratory at Cambridge University. This lets us observe wood on a nanometer scale – seeing structures that are over 6,000 times smaller than a single strand of human hair – while preserving the wood’s moisture to give a more accurate impression of what the wood looks like while the tree is alive.
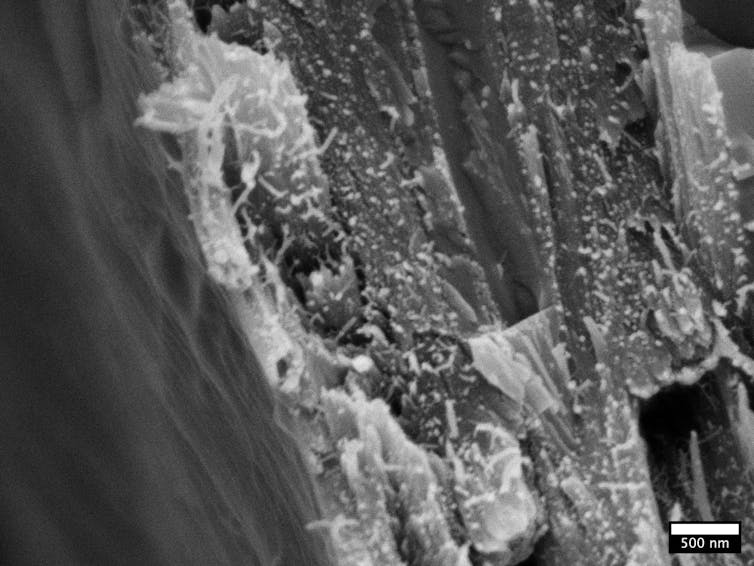
The evolution of wood structure
We studied various trees in the Cambridge University Botanic Garden to understand the evolution of wood structures. We collected living samples of plants that represent key milestones in evolutionary history. These plants are a short walk to the microscope, enabling us to examine the samples without them drying out.
We found that the size of the macrofibril, a fibre composed mainly of cellulose, which is the basic chemical building block of wood and gives plants the strength to grow tall, varies significantly between hardwoods and softwoods. In hardwoods, like oak and maple, the macrofibril measures about 16 nanometers (nm) in diameter, while in softwoods like pine and spruce, it’s about 28 nm. These differences could explain why softwoods and hardwoods are different and may help us figure out why some kinds of wood are better at storing carbon than others.
Understanding how wood evolved can help us identify and exploit plants that might mitigate climate change. The tulip tree alone does not tell us this, so we went further back in time and examined basal angiosperms, a group of rare and ancient flowering plants that still exist as remnants of the earliest stages of plant evolution. One member of this group is Amborella trichopoda, which has the larger 28 nm macrofibrils, which suggests hardwood macrofibrils came about later than softwoods.
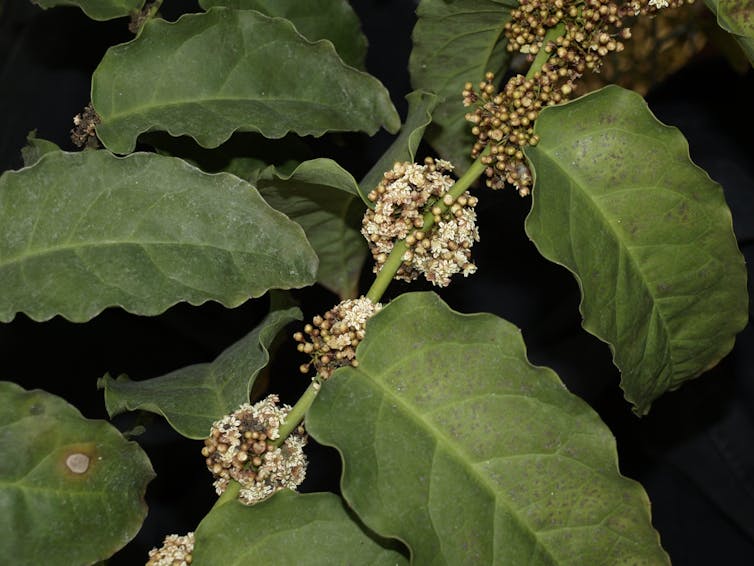
But when exactly did it happen?
To answer this question, we explored the magnolia family, including the purple-flowered Magnolia liliiflora, which are some of the oldest surviving flowering plants that are known for their ornamental beauty. The ones we tested have hardwood-like macrofibrils with a diameter of 15-16 nm, meaning the switch from softwood to hardwood likely occurred during the evolution of the magnolias.
The tulip tree is a close relative of the magnolias, but its wood does not fit neatly into the softwood or hardwood categories. Instead, its macrofibrils had a diameter of about 22 nm – in the middle of the range between hardwoods and softwoods This intermediate structure was completely unexpected and led us to classify tulip tree wood as “midwood”, a new category entirely.
Midwood: a super carbon accumulator?
Why do tulip trees have this unique wood type? We can’t say for certain, but we believe it’s related to the evolutionary pressures these trees faced millions of years ago.
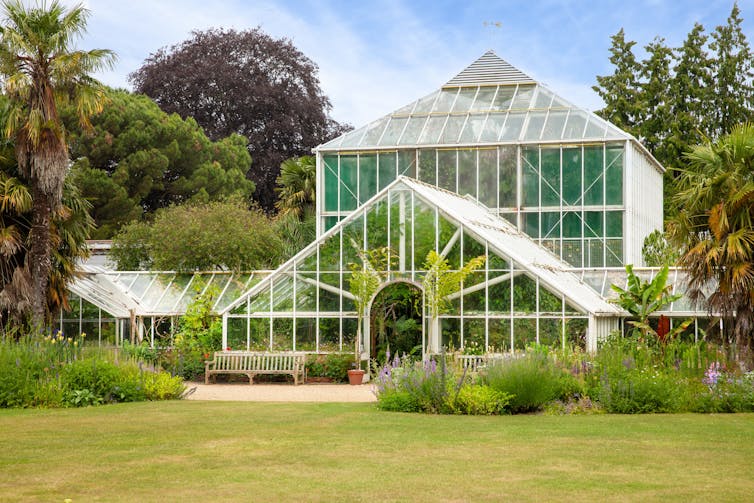
When tulip trees first evolved, atmospheric CO₂ levels were falling from about 1,000 parts per million (ppm) to 500 ppm. This reduction in available CO₂ may have driven tulip trees to develop a more efficient method of carbon storage, leading to their unique macrofibril structure. Today, this adaptation likely contributes to their exceptional ability to sequester carbon.
We can no longer assume, when looking at a previously unstudied tree, that it falls into the same two categories (softwood or hardwood) scientists have placed trees in for years. The tulip tree, with its midwood structure, corresponds with a “carbon-hungry” attitude. We are now looking at whether its seemingly unique wood structure is the sole reason it is king of carbon capture, and we are widening our search to find out if there are any more midwood trees – or even more new wood types out there.
These findings underscore the importance of botanical research and the role that collections, such as those at the Cambridge University Botanic Garden, play in uncovering new insights in plant science. Next time you visit a botanic garden, remember that there are still many mysteries hidden in the plant kingdom, waiting to be discovered.
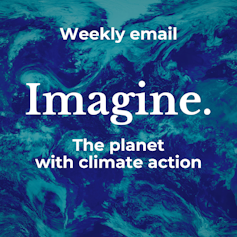
Don’t have time to read about climate change as much as you’d like?
Get our award-winning weekly roundup in your inbox instead. Every Wednesday, The Conversation’s environment editor writes Imagine, a short email that goes a little deeper into just one climate issue. Join the 35,000+ readers who’ve subscribed so far.

Raymond Wightman is employed at the Sainsbury Laboratory Cambridge University which receives core funding from The Gatsby Charitable Foundation.
Jan Łyczakowski receives funding from National Science Centre Poland.
This article was originally published on The Conversation. Read the original article.