This story originally appeared in Hakai Magazine and is republished here as part of Covering Climate Now, a global journalism collaboration strengthening coverage of the climate story
It’s the world’s most vulnerable glacier and key to the stability of the West Antarctic Ice Sheet, yet we’re only now getting to know Thwaites Glacier. What took us so long?
RAM! The bright red hull of the US Coast Guard icebreaker Glacier slammed onto the ice. Every rivet of the 95-metre-long ship shuddered with the impact. It was 1985, and the researchers aboard were sailing through one of the world’s most remote places: the Amundsen Sea, Antarctica. A graduate student onboard, Jill Singer, got to try her hand at breaking ice during the voyage. “You push the throttle or thrusters up,” she explains. “It would lift the front of the Glacier out of the water enough to drop down on the ice and break it.” RAM! After several days of charging at sea ice with bone-aching resolve, the Glacier broke through.
“[We] broke into … a beautifully calm, ice-free sea, the eye in a hurricane of ice,” glaciologist Terry Hughes later wrote of the moment — one he had been dreaming of since at least the 1970s when he first started worrying about glacial collapse.
The Glacier became the first ship to sail into Pine Island Bay.
The Intergovernmental Panel on Climate Change predicts sea levels will rise almost half a metre by 2100. That water will displace several million people on coastlines around the world. Much of the water will come from the region around Pine Island Bay. Specifically, it will come from what’s been dubbed the “Doomsday Glacier”: Thwaites, one of our planet’s largest glaciers, which is roughly as extensive as Great Britain.
Glaciers form when snow is compacted into ice over hundreds of years. As the weight of new snow and ice presses down, the ice beneath starts to flow like a river. Thwaites is an outlet glacier, meaning it flows all the way to the ocean. There, its coastal edge stretches 120 kilometres in a dazzling white wall of ice that looms up to 40 metres above the surface of the ocean and reaches over 200 metres deep.
Thwaites and its neighbour Pine Island Glacier drain about one-third of the West Antarctic Ice Sheet — the ice sheet extending west from the natural dividing line of the Transantarctic Mountains. The two glaciers are breaking up into icebergs far more quickly than new ice can be created. Already they contribute five percent of annual sea level rise, or roughly 0.18 millimetres annually: the equivalent of dumping over 20 million Olympic-sized swimming pools into the ocean each year. And if Thwaites collapses, its shape and location mean the rest of the West Antarctic Ice Sheet could go with it. All told, that’s enough water to raise sea levels by over three metres, redrawing coastlines and transforming the planet we know.
Researchers like Hughes have been raising concerns for nearly 50 years about the glaciers that flow into Pine Island Bay and the surrounding Amundsen Sea embayment. Yet coordinated international research of the region only took off in 2018, with the formation of the International Thwaites Glacier Collaboration. Today, the potential collapse of Thwaites Glacier is among the largest environmental threats to global civilisation — and we’ve barely begun to understand it. What took us so long?
As it turns out, Pine Island Bay is one of the hardest places in the world to reach. The story of how we know what we know about Thwaites is also a story of the challenges — and triumphs — of science at the bottom of the world.
Pine Island Bay is a small indent in the coast of West Antarctica. It feeds into the stormy and ice-choked Amundsen Sea — the only sector of Antarctica that no nation has bothered to claim. It’s remote even for Antarctica: the closest permanently occupied research station is 1500 kilometres away.
The first ship known to have reached the Amundsen Sea was commanded by Captain James Cook. In January 1773, he and his crew on the HMS Resolution were the first humans to cross the Antarctic Circle, the invisible line of latitude at 66° south. Cook had been sent south by the British government to determine whether land existed below Australia, a region of longstanding curiosity to members of England’s leading scientific institution, the Royal Society.
A year later, the Resolution again crossed the Antarctic Circle but was stopped short by what Cook described as an “immence Icefield … so close packed together that nothing could enter it.” The ship was at S 71°10′, W 106°54′. According to legend, young midshipman George Vancouver clambered to the bowsprit and waved his hat over the icy waters, declaring himself the southernmost human in history. The ship’s coordinates became known as Cook’s ne plus ultra — Latin for “no more beyond.”
When Cook turned back, the Resolution was less than 300 kilometres directly north of Thwaites Glacier. It’s a short distance by today’s standards, yet when a modern edition of Cook’s journals was published in 1971, his ne plus ultra in that region remained unbroken. In 200 years, no one had sailed farther south into the Amundsen Sea than Cook.
Pine Island Bay was first seen not by sea, but by air. In the 1940s, the US Navy organised Operation Highjump, which sent aircraft carriers to map different parts of the Antarctic coast. The USS Pine Island, named for Pine Island Sound in Florida, was sent to the Amundsen Sea. But the Pine Island never entered Pine Island Bay. Instead, its aircraft returned with the first aerial views of the embayment.
With little incentive to brave the harsh conditions of the Amundsen Sea, the US Navy and Coast Guard focused on other Antarctic regions over the following decades. By 1980, Pine Island Bay remained the largest unmapped coastal area below the Antarctic Circle.
Around that time, though, Hughes had begun to ask questions about the region. The glaciologist, who called himself a “cowboy scientist”, was outspoken and had a flair for making dramatic statements that could distract from the science. But he was also respected as a keen theorist whose papers bristled with mathematical equations predicting the behavior of glacial ice under various conditions.
As early as the 1970s, Hughes was concerned with two problems not then widely studied: a warming planet and the potential collapse of the West Antarctic Ice Sheet. He had become involved in a major project to reconstruct the growth and collapse of ice sheets during the last ice age. This project had drawn his attention to Pine Island Bay as a coastal area where the outer edge of glaciers might calve or break apart into icebergs with especial rapidity.
Hughes had noticed that satellite images showed a surprisingly short glacial shelf. Why did the glacial ice not extend all the way across the bay? Outlet glaciers generally flow into an ice shelf, a floating expanse of ice reaching over the water. In certain cases, this floating shelf can buttress and protect the “grounded” inland ice that rests directly on bedrock. A similarly sized glacier in East Antarctica, David Glacier, flows for 100 kilometres over the ocean. Pine Island Glacier’s floating ice, by contrast, is half that length.
At the time, scientists knew the planet was slowly warming, but many believed Antarctica was safe: simply put, it was too cold to fail. Hughes wasn’t so sure. He worried that the lack of a large floating ice shelf in Pine Island Bay indicated instability among the nearby glaciers, which reach deep into the heart of the West Antarctic Ice Sheet.
In 1981, he dubbed Pine Island and Thwaites Glaciers the “weak underbelly” of West Antarctica: glaciers whose weakening could trigger the catastrophic collapse of the West Antarctic Ice Sheet. And he did mean catastrophic. Most of West Antarctica might be open water within 500 years; the oceans could rise by over a metre in one human lifetime. With the bombast that raised eyebrows among his colleagues, Hughes titled one of his research papers “Deluge II and the continent of doom: rising sea level and collapsing Antarctic ice.”
When Hughes sailed past Cook’s ne plus ultra and entered Pine Island Bay in 1985, he was a guest onboard with no official funding. Yet he hoped to learn more about Pine Island Glacier — particularly, whether it was flowing too fast to be stable. Ice and wind conditions in the bay were so bad, though, he was unable to collect useful data.
For the National Science Foundation (NSF), which had funded the research cruise, curiosity alone couldn’t justify the fuel needed to ram ice day and night on the taxpayer’s dime. If there were a glaciological fire smoldering in Pine Island Bay, as Hughes argued, NSF needed to see the match. Hughes hadn’t found it.
In fact, early satellite data was casting doubt on Hughes’s theories. In 1972, fresh from the space race, NASA and the US Geological Survey had launched the first Earth Resources Technology Satellite (now renamed Landsat).
“Remote sensing has revolutionised glaciology,” says glaciologist Karen Alley. “It’s not that long ago that nobody even had a picture of the whole continent,” she adds, referring to Antarctica. “And now we have continent-wide ice thickness and flow velocity.”
Throughout the 1980s and early ’90s, studies based on Landsat satellite images suggested that Pine Island Glacier was not only stable, it was actually gaining ice: 50 gigatonnes of ice per year, according to one estimate. It was fattening up to match its nickname, the PIG. Thwaites Glacier — an afterthought in these studies — was apparently growing, too.
It seemed Pine Island Bay shouldn’t be considered an area of concern. Hughes wasn’t convinced. The bay’s lack of floating ice shelves still raised his suspicions. Without data to support his theories, though, there was little he could do.
How did the studies using Landsat get it so wrong? “There’s a lot it doesn’t do,” Alley explains. Landsat specialises in capturing data at the surface; it turns out the crucial information Hughes needed was hidden beneath the ice, where Landsat couldn’t reach. But a different type of satellite technology was about to turn glaciology on its head.
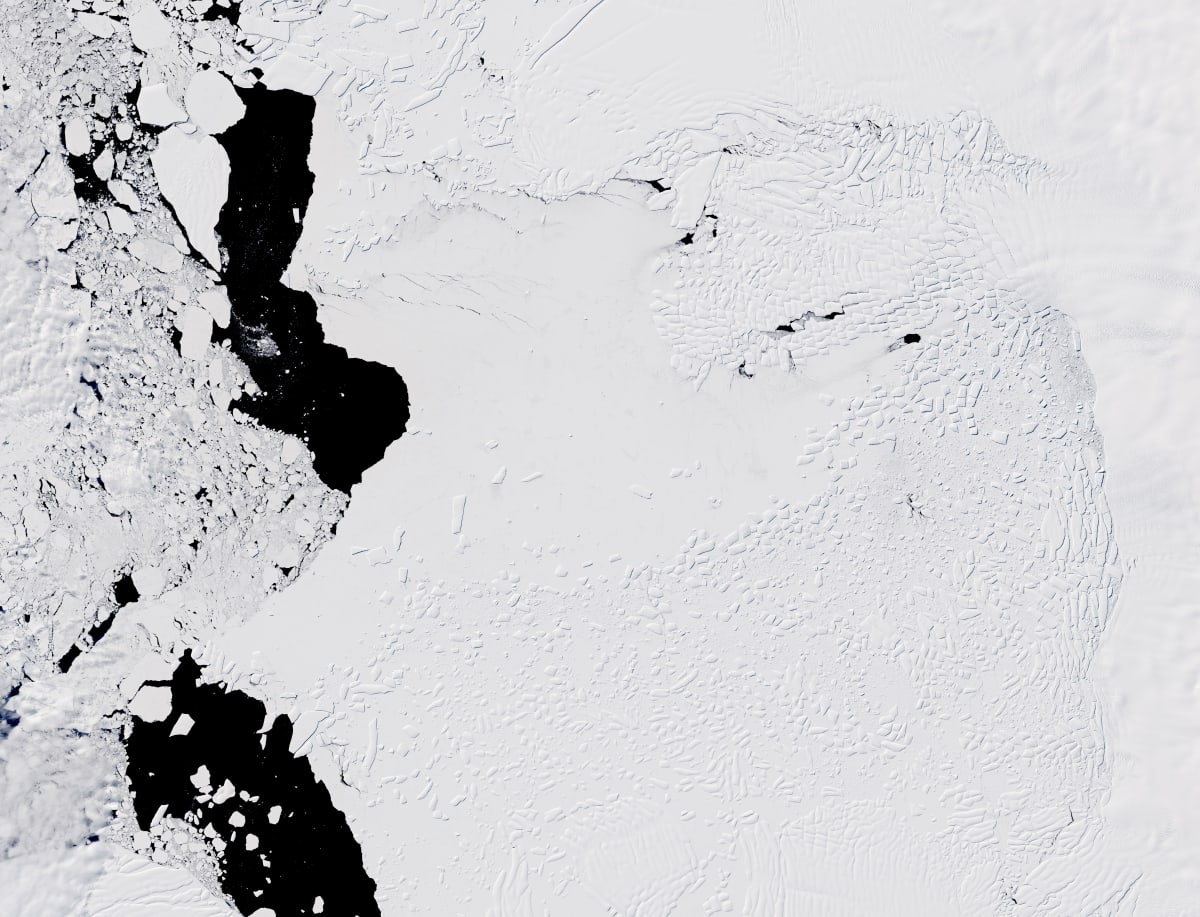
In 1991, the European Space Agency launched the first European Remote Sensing satellite. It carried instruments for a new technique called radar interferometry. Eric Rignot, a glaciologist at the University of California, saw the technique’s potential, particularly after following its use on a NASA space shuttle a few years later.
Using radar interferometry, Rignot was able to pinpoint facts about the glaciers that had once been invisible. He could see deformations in the ice with accuracy up to one millimetre. He could read ice movement not year to year, not even month to month, but by the hour. Most importantly, he could locate the grounding line.
When it comes to glacial collapse, the action is at the grounding line, where a glacier lifts up from the bedrock and begins to float over the ocean. It’s also where ocean water gnaws at that glacier’s base, loosening it from the bedrock. Much of West Antarctica’s ice is grounded well below sea level, in a bowl-shaped marine basin. Warmer ocean water can flow downhill into this bowl, melting the ice at the grounding line and causing it to move inland. When floating ice melts, it doesn’t change sea level: it’s already displacing ocean water, like ice cubes in a glass of water. But when the grounding line retreats, once-grounded ice melts. That does increase sea level and also destabilises the glacier: the ice is thinned out from below and breaks apart more easily.
In the sloping marine basin beneath Thwaites, the wall of submerged ice stretches over 1000 metres deep, giving the water an enormous surface area to work on. The water can push the grounding line inland rapidly, only slowing if it encounters bedrock that is elevated — say, an underwater ridge or mountain that “pins” the ice. Thwaites Glacier has one such pinning point, about 40 kilometres behind the current grounding line. That pinning point sustains pressure against the glacier’s interior, like a flying buttress pressing against the wall of a cathedral. If the ice shelf is loosened from that pinning point, the glacier may pour its ice out even more rapidly.
Researchers can’t tell how fast the grounding line is retreating if they don’t know where it is. With this vital information, Rignot was getting melt rates orders of magnitude higher than ever seen before. He calculated that a major glacier in Greenland was melting by up to 20 metres per year. (Previous data had suggested that even the most vulnerable glaciers melted about 10 to 20 centimetres annually.) Rignot knew the figures would look wild to many of his colleagues. “Woah! Maybe my data is bad,” he thought, “but the evidence is piling up that these melt rates are way bigger.” He received pushback throughout the 1990s. It was hard for many to believe glaciers could disappear that fast. What could destroy a glacier so quickly?
The answer arose from an unexpected source. An oceanographer at Columbia University in the City of New York, Stanley Jacobs, thought the ocean might be involved. He knew of Hughes’s work and in 1991 had published a paper urging “icebreaker penetration and detailed oceanographic sampling” of the “largely unknown” Amundsen Sea. He wanted to take a ship back to Pine Island Bay.
The ocean’s importance may seem obvious now, but at the time researchers were more focused on flow velocity than on what happened when the glacier reached the ocean. In glaciology, Rignot says, few thought the ocean could matter: “It wasn’t on the horizon.”
The ocean is notoriously difficult to study. It’s expensive and dangerous, even in the best conditions. Rignot’s improved satellite data was so good that he mostly worked from his office in California. Where the ocean was concerned, though, satellites weren’t enough. Satellites can give temperatures only at the surface, where ice melt and subzero winds make polar water very cold; they can’t reach the deeper warm water.
“To get data in the ocean, you have to go there,” Rignot says. In 1994, Jacobs did just that.
“We managed to get the ship into Pine Island Bay, which was obviously the place above all others I wanted to get, and the observations changed our thinking about the region.” — Adrian Jenkins
No one had broken through the ice around Pine Island Bay since the voyage of the Glacier a decade earlier. “We were going into essentially uncharted waters,” recalls the oceanographer Adrian Jenkins, who joined Jacobs onboard. “No one knew where the edge of the continental shelf was — it was mismapped.”
Their cruise started in the more accessible waters of the Ross Sea, south of New Zealand. There, the team found extremely cold ocean water — about -2.2 °C, well below the freezing point of fresh water. As they followed the coast toward Pine Island Bay, though, something changed. They entered Sulzberger Bay, around the corner from the Ross Sea, and found water at 0 °C.
The water got warmer still as they approached their goal. “We managed to get the ship into Pine Island Bay, which was obviously the place above all others I wanted to get,” Jenkins recalls. “And the observations changed our thinking about the region.” Startled by the warm temperatures, he taught himself the scientific software MATLAB onboard and coded up some rough estimates. “I found these really high melt rates, like 100 metres per year — I thought way too high, way too high,” Jenkins recalls. “I thought there must be something wrong; I spent the next 10 years trying to figure out what. Turns out it’s not so much of an overestimate as I thought.”
Initially, though, the team had trouble publishing their results. A paper they submitted to a major publication was rejected without outside review, dismissed as not being of sufficiently broad importance.
Rignot became a key ally. His work had focused on Greenland, but he knew of Hughes’s seemingly niche obsession with Pine Island Bay. He was intrigued by Jenkins’s high melt rates, which aligned with the figures Rignot had found in Greenland. The warm water that Jacobs and Jenkins had discovered in Pine Island Bay brought the whole picture together.
“I had heard this theory on the instability of West Antarctica,” Rignot recalls. He began looking at radar interferometry data on Pine Island Glacier’s grounding line, and “Boom! It was flashing on my screen. Something big was happening here.” He took almost two years to publish his calculations: “I wanted to be darn sure that what I was seeing was real,” he says. “Because if it was real, that was very significant.”
At a conference in 1997, Rignot presented findings indicating that Pine Island Glacier’s grounding line was retreating by roughly 1.2 kilometres per year.
“This was where Eric’s work set the world alight,” Jenkins remarks. Jenkins, Jacobs, and their colleagues had shown that the glaciers were melting faster than previously thought, due to the warm ocean. But melting can still be a relatively stable process and not always a problem. The danger arises when the grounding line retreats, giving warm ocean water ever greater access to the interior of the ice sheet. In these cases, the glacier may not melt slowly like an ice cube — it may collapse like a cathedral.
Today, Rignot’s calculation holds: researchers believe Pine Island Glacier’s grounding line retreated by around one kilometre per year in the two decades before 2011 (it seems to have slowed down recently). Thwaites continues to retreat around the same rate of one kilometre a year and loses around 37 gigatonnes of ice annually. (That’s enough to bury the contiguous United States five millimetres deep in ice every year.) When Hughes started looking into Pine Island Bay in the 1980s, he may not have understood the mechanisms, but he was right to worry.
Changing the direction of Antarctic research is like driving an icebreaker, though. It’s slow and expensive. After the 1994 cruise, it took NSF six years to send a ship back to Pine Island Bay, and the ice was so thick on the first attempt (in 2000) that Jacobs couldn’t get close to Pine Island Glacier. In the meantime, he and Jenkins continued publishing with Rignot to strengthen their case that warm water was rapidly shifting the grounding line.
Finally, in 2009, their ship broke through. It had been 15 years since anyone had sailed these waters. Now, though, they had new cutting-edge technology: Autosub3, a fully automated underwater drone. Autosub3 confirmed Jacobs’s original suspicions that the ocean was the culprit: a deep band of warm water, called Circumpolar Deep Water, was getting under the glacial shelf. It had already eaten an enormous cavity under Pine Island Glacier.
The next generation of polar researchers responded to these developments at the bottom of the world. David Holland, a Canadian mathematician interested in modelling ocean-water-air interactions, had been on the second plane to land atop Pine Island Glacier in 2007. He knew that ocean currents respond principally to wind and other atmospheric patterns, and had developed a sophisticated weather station to find out what the atmosphere was up to. He and two assistants camped out on Pine Island Glacier five summers in a row before they shifted their focus to Thwaites.
They were still figuring out where to focus their research, he explains. Building on previous work, Holland’s team started at Pine Island. But, he says, “while we were there, we thought, Shouldn’t we be next door at Thwaites?”
The storms around Thwaites and its vast extent make it especially difficult to study. In 2004, a joint project between the United States and the United Kingdom had included the first systematic airborne survey of the topography beneath Thwaites, revealing patterns of ice flow into the glacier’s interior and its connection to the surrounding ice sheet. The scope of its possible impact was becoming clear.
Thwaites is larger than Pine Island Glacier — much, much larger. It has a wide front — over 120 kilometres — and its base slopes steeply down to nearly 1000 metres below sea level. These dimensions give the warm ocean water a lot of ice to work with. Moreover, Thwaites’s catchment basin, meaning the ice that flows into the glacier, is around 700 kilometres long, the distance from Boston, Massachusetts, to Washington, DC. In short, it’s the perfect candidate for collapse on a massive scale. Today, Thwaites contributes more sea level rise than Pine Island Glacier by a factor of four to one.
For decades, though, the focus had been on Pine Island Glacier. The two glaciers are neighbours — but on an Antarctic scale of over 50 kilometres of thick sea ice between the most accessible ice fronts. In fact, since earlier cruises had been so intent on reaching Pine Island Glacier, it’s possible that nobody saw Thwaites from shipboard until 2019. And as Holland found when he sailed there in January 2022, the glacier’s disintegration is making it even harder to reach.
Headlines in December 2021 announced that the Thwaites ice shelf might “shatter like a car windscreen” within five years. That’s hard to say for sure. We do know that the floating ice shelf acts like a buttress, keeping Thwaites’s inland, grounded ice stable. We also know that the ice shelf is fracturing into icebergs at unprecedented rates. If the ocean drives the grounding line back too far, it can cause runaway melting.
Thwaites is a cork in the bottle of West Antarctica. Its vast size and central position mean that its collapse could trigger a reaction across the entire West Antarctic Ice Sheet. It’s possible this happened around 125,000 years ago, when sea levels were about six to nine metres higher than today. West Antarctica won’t fall apart overnight. It might take a few hundred years. But if it does happen — as many researchers fear it will — it will redraw global coastlines.
Holland sees the planet in terms of a simple principle: “play with the atmosphere, expect change.”
Unusually warm ocean currents are melting the ice. Those currents are driven by shifting wind patterns: stronger winds displace cold surface water, allowing deep warmer water to rise up and pour over the continental shelf into the marine basin beneath the glaciers. The winds, in turn, respond to one thing: changes in air temperature. And those changes are caused by greenhouse gas emissions.
In short, Holland says, “the winds will change the ocean, the ocean will melt Antarctica — and the water is coming to visit you.” There is evidence that atmospheric changes can raise sea levels by several metres within the space of a century. But the systems are so complex that they’re hard to predict. We have apps on our phone to tell us the weather tomorrow, Holland remarks, but we’re a long way from having such apps for the ocean or ice sheets. In fact, the Intergovernmental Panel on Climate Change asserts that predicting the “dynamic contribution” of ice sheets “remains the key uncertainty” in sea level rise projections.
Science is a fallible, communal human process; it moves by slow self-correction, threading its way through uncertainties like a ship among icebergs. In Pine Island Bay, where the ocean charts themselves are still being updated, precision is vital. But precision takes time, and Thwaites’s time seems to dwindle with each new study.