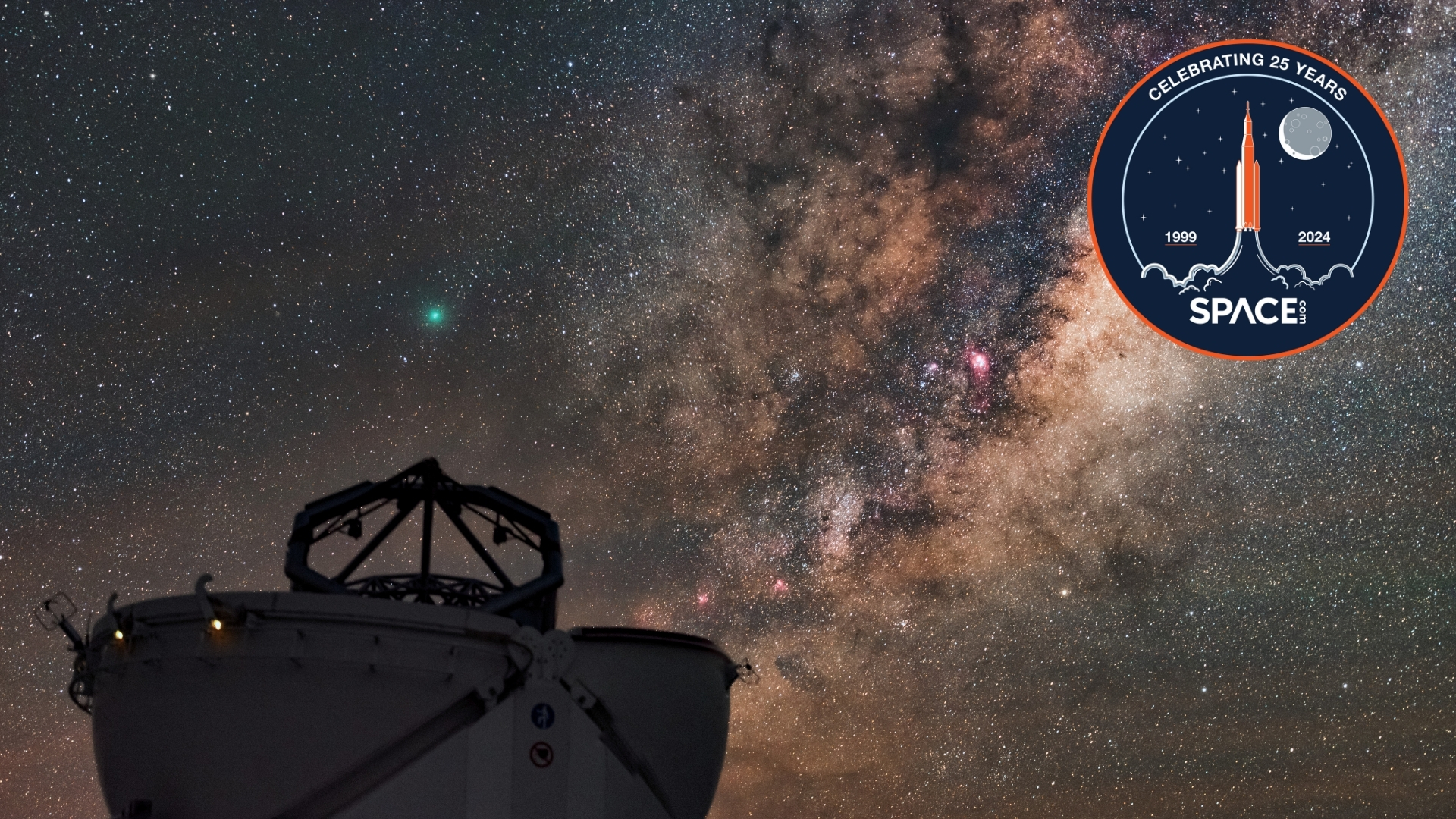
The past 25 years have, if anything, been the era of the mega-telescope, an era that began in the 1990s. Until then, the largest optical telescope in the Western Hemisphere had been the 200-inch (5.1-meter) Hale Telescope, built in 1949 on Mount Palomar in California and used by Edwin Hubble in the years before his death. A Soviet telescope called the BTA-6 beat it by one meter (39 inches) in 1975 — but, for the next 40 years, the largest ground-based telescopes were all built in the 4m to 5m (157 in to 197 in) class.
There were reasons for why telescopes hit a glass ceiling — pun intended — for over four decades. One of those reasons was that the glass mirrors of giant telescopes were typically incredibly heavy, built out of thick glass to render them impervious to gusts of wind, thermal expansion or the shake of an earthquake. Make the mirrors any larger, and they would sag under their own weight, deforming the shape of the mirror and making the telescope's vision blurry.
Then, in the 1980s, more lightweight glass mirror technology was developed, principally by John Hill and J. Roger Angel at the University of Arizona, Tucson. Rather than use a solid chunk of glass to make a telescope's mirror, they built a lightweight honeycomb structure from ceramic fiber, added broken chunks of glass, then melted the glass in a furnace while spinning it on a turntable. The combination of centrifugal force and gravity created a parabolic shape to the finished mirror that was both lightweight and stiff, thanks to the interior honeycomb structure.
Check out a list of Space.com's special 25th anniversary week stories in our hub linked here!
By the 1990s, it was possible to make spin-cast mirrors of up to 8.4 m (331 in) in diameter, and suddenly, much larger observatories were possible to construct. Yet, even before observatories were able to make use of this technology, several other observatories had circumvented the limitations of large mirrors to use a technology of the future: segmented mirrors formed from smaller hexagonal sections that didn't suffer the same problem of heavy weight and mirror sag; they were also easily transportable, enabling much larger telescope apertures.
The giant 10 m (394 in) Keck I telescope on an inactive volcano, Mauna Kea, in Hawaii was the first to use this segmented technology in 1993, offering twice the aperture of the Hale Telescope. Keck II followed in 1996, as did the 9.8 m (386 in) Hobby-Eberly Telescope at McDonald Observatory in Texas that also used a segmented design.
Their construction kicked off the race to build giant ground-based telescopes in the 8–10 m (314–394 in) class. Using spun-cast mirrors, the 8 m (315 in) Japanese Subaru telescope saw first light on Mauna Kea in 1999, the Gemini North and South 8.1 m (319 in) telescopes in 1999 and 2001 respectively, and the four 8.2 m (323 in) unit telescopes of the European Southern Observatory's Very Large Telescope (VLT) on Cerro Paranal in Chile, which individually saw first light between 1998 and 2000, came about just around the time that Space.com itself was being launched.
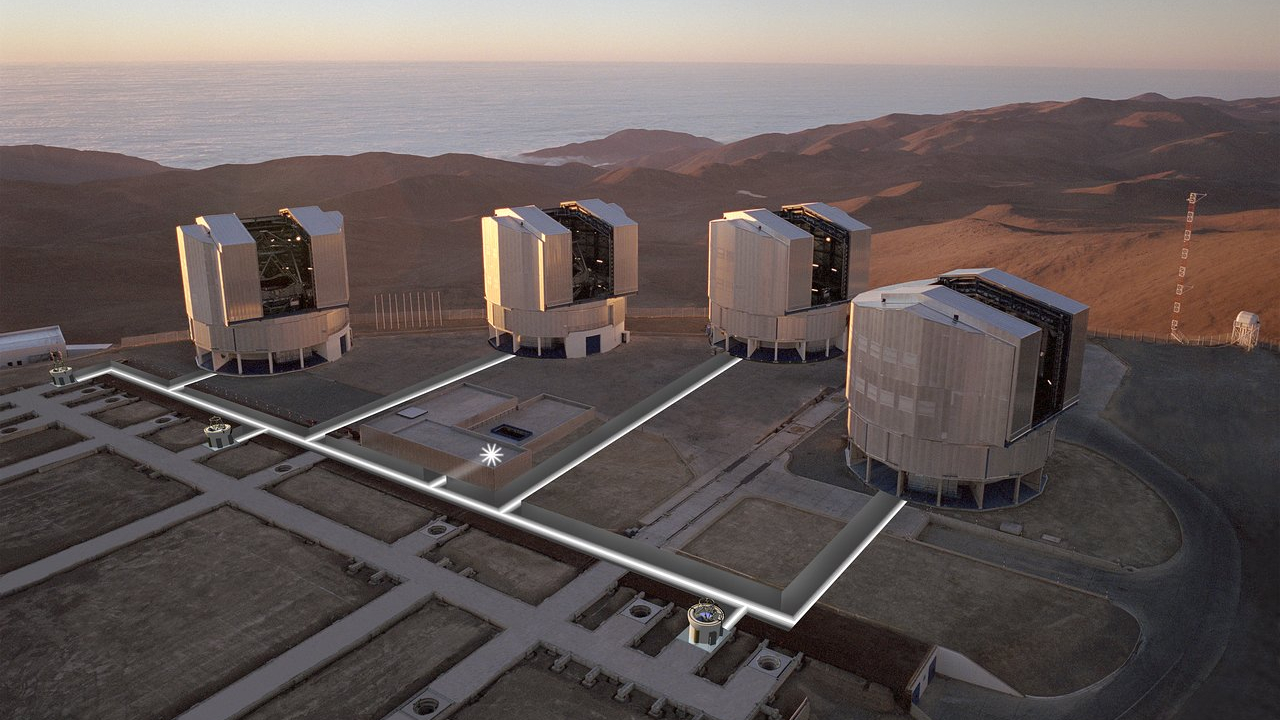
Several slightly large telescopes have come on the scene since: the Large Binocular Telescope with two 8.4 m (331 in) mirrors in Arizona in 2004, the 9.2 m (362 in) South African Large Telescope in 2005, and the giant 10.4 m (409 in) Gran Telescopio Canarias in the Canary Islands in 2006, which is currently the largest optical telescope in the world.
It won't remain so for much longer.
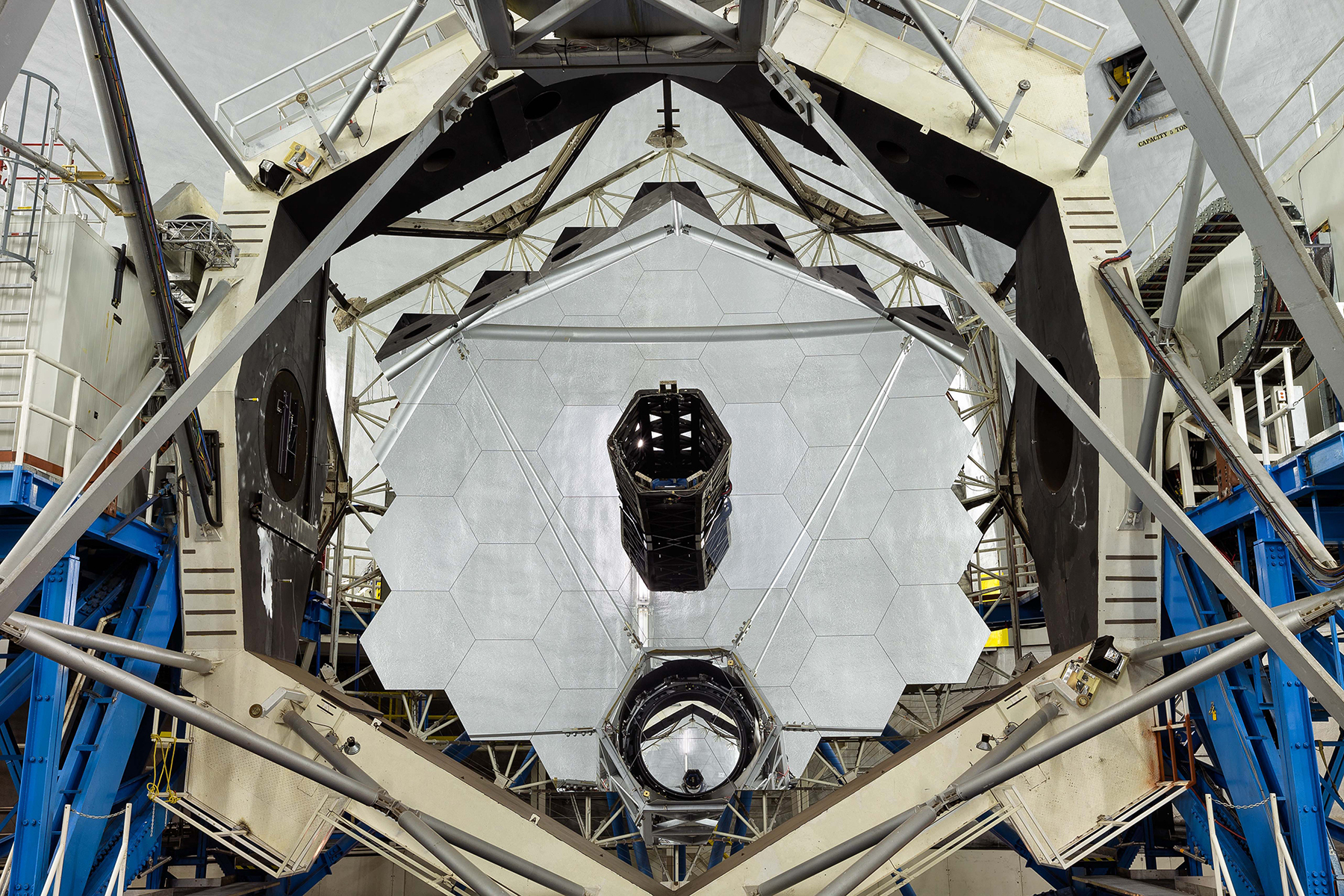
In 2002, astronomers used the W. M. Keck Observatory to confirm the existence of a 4.1-million-solar-mass black hole at the center of the Milky Way galaxy, using the motions of stars close to it to measure its mass. Subsequently, the VLT has been involved in a multi-decade study to better track the orbits of these stars, showing how the extreme gravity of the supermassive black hole alters those orbits, in accordance with Einstein's theory of general relativity. The work of both Keck and the VLT resulted in astronomers Reinhard Genzel and Andrea Ghez winning the 2020 Nobel Prize in Physics.
The Gran Telescopio Canarias was the last of the 8–10 m (315-394 in) class to be built. Since then, astronomers' attention has been on the next breed of extremely large telescope, with apertures dozens of meters across. The European Southern Observatory's 39-meter (128-foot) Extremely Large Telescope (ELT) is under construction atop the Cerro Armazones mountain in Chile, with the intention of seeing first light by the end of this decade. Its contemporaries will be one of, if not both, the Thirty Meter Telescope (TMT) and the Grand Magellan Telescope (GMT), the latter of which will sport seven 8.4-meter-wide mirrors giving a total aperture 25.4 meters across.
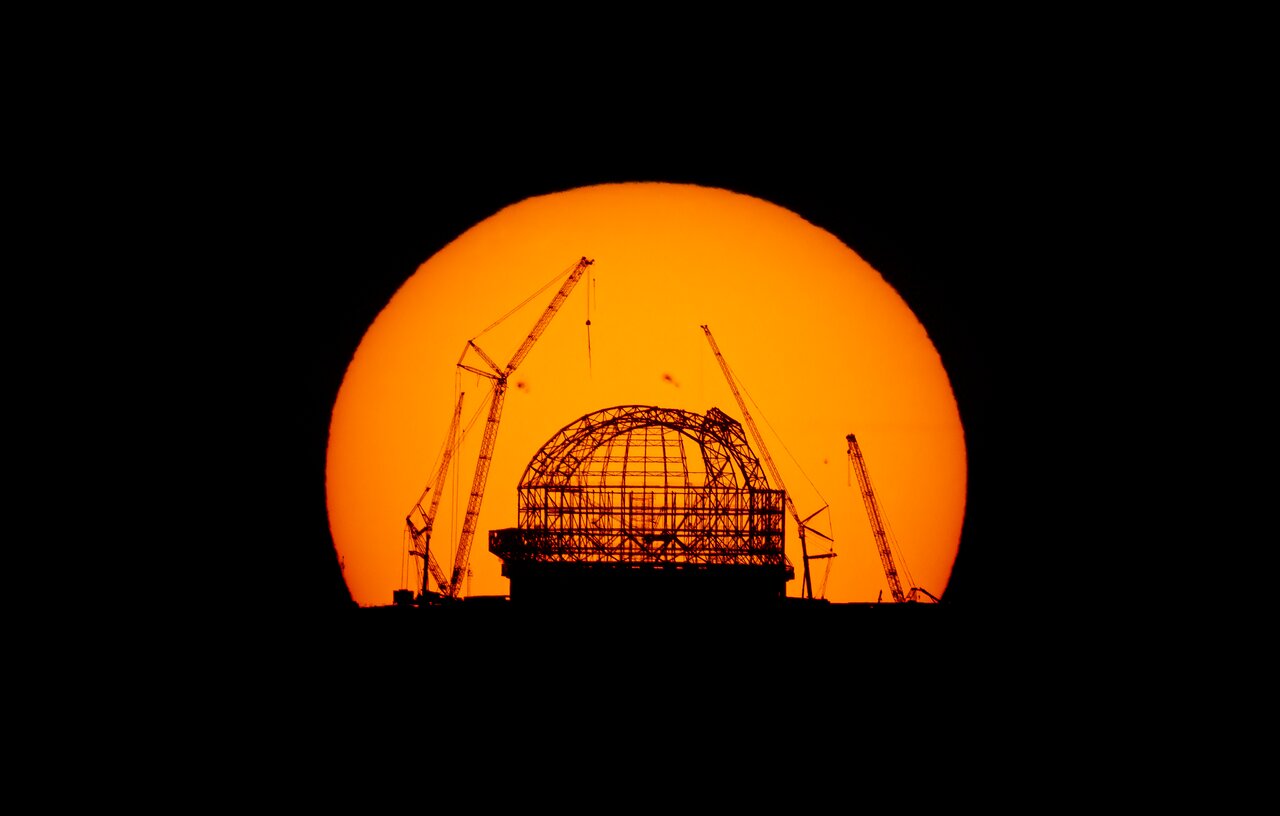
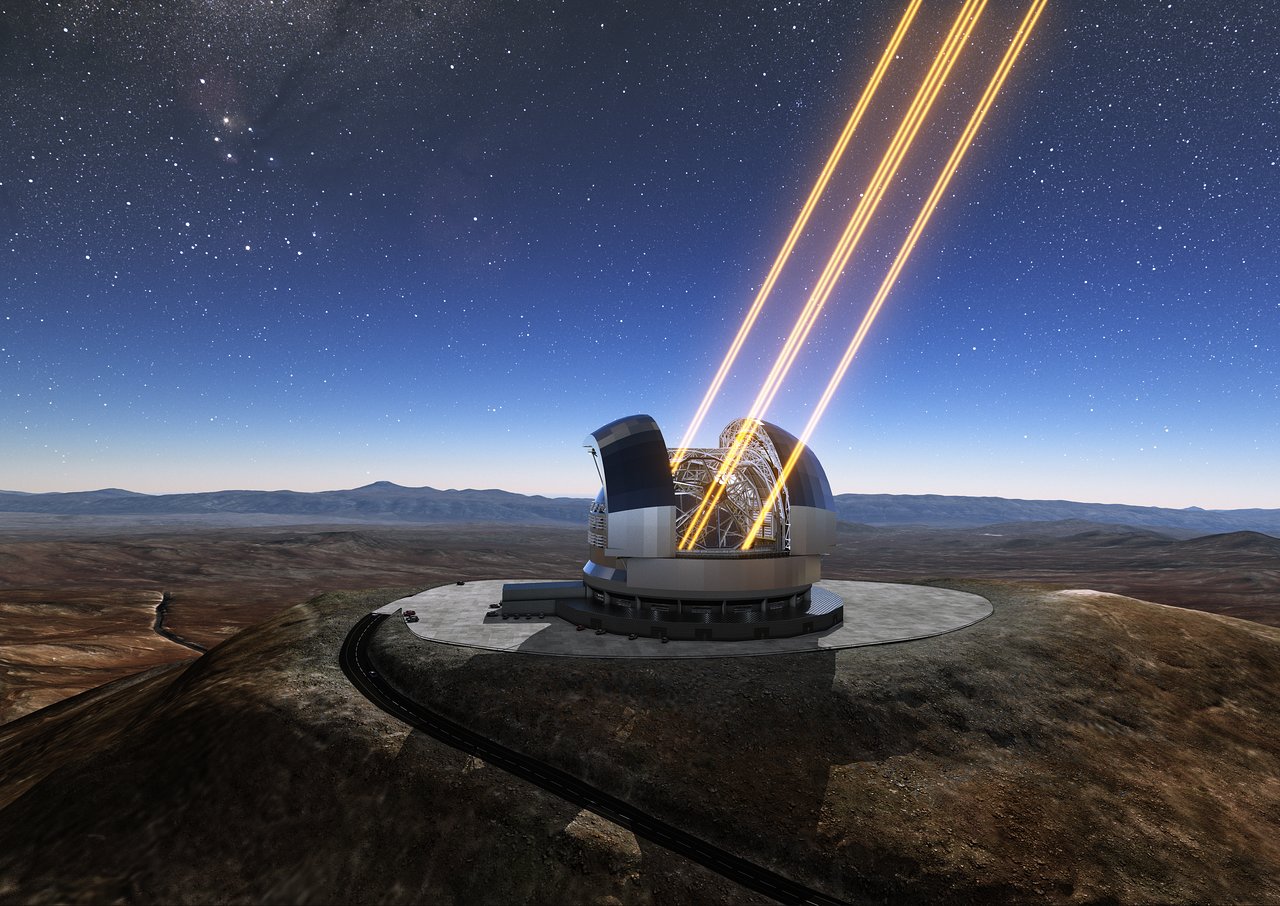
Hunting for exoplanets
When gravitational waves were detected from a collision between two neutron stars in Aug. 2017, the afterglow of the explosion, called a kilonova, was spotted in a galaxy 140 million light-years away. Many of the world's largest observatories, including SALT in South Africa, Keck and Gemini leapt into action to characterize this afterglow. It was the first time such an event had been seen in virtually every realm of the electromagnetic spectrum, including gamma-rays, X-rays, ultraviolet, optical, infrared, radio and gravitational waves.
Phew! That's a lot of mega-telescopes since the 1990s. Is it a coincidence that the 1990s also saw the beginning of one of the biggest growth areas in astronomy, namely the search for and characterization of exoplanets?
No it isn't, according to Aprajita Verma, who is a project scientist for the Extremely Large Telescope at the University of Oxford.
"Over the past 20 or 30 years, what has really pushed us towards the higher spatial resolution and higher sensitivity that larger apertures bring has been the discovery of extrasolar planets," Verma told Space.com.
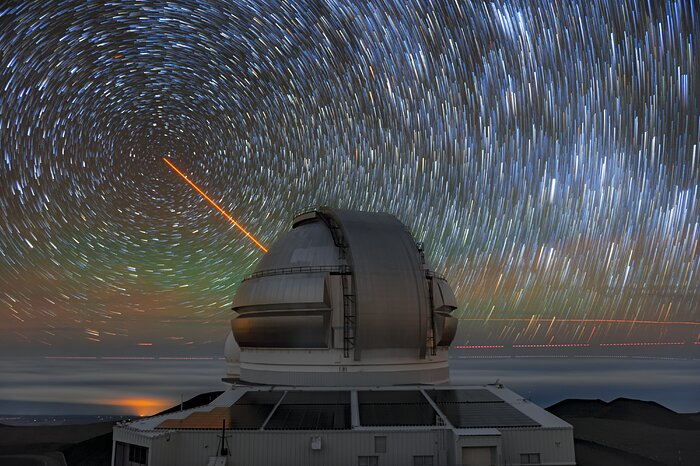
This milestone was achieved in 2004 and confirmed in 2005 by astronomers using the Very Large Telescope, who imaged a planet with between 5 and 6 times the mass of Jupiter, called 2M1207b, orbiting a brown dwarf 230 light years away.
The first exoplanets around sun-like stars were discovered in 1995. Large telescopes are not needed to find more exoplanets — far smaller telescopes are capable of that. What is needed, however, is large apertures with enough sensitivity to begin characterizing these alien worlds, first through spectroscopy of their atmospheres and increasingly through direct imaging; in other words, we need to take clear pictures of exoplanets. In 2005, the first-ever direct image of an exoplanet was taken by the VLT's NACO instrument, which is a combination of its Nasmyth Adaptive Optics System (NAOS) and its Near-Infrared Imager and Spectrograph (CONICA).
Currently, the VLT uses its Spectro-Polarimetric High-contrast Exoplanet Research (SPHERE) instrument, which is specifically designed to image exoplanets that are young, warm and large — like baby Jupiters, if you will. It uses a device called a coronagraph, so named because it has its origins in solar astronomers using it to block out the glare of the sun to see the solar corona, usually only visible during total solar eclipses. The coronagraph blocks out the light of a planet's parent star, so that SPHERE can detect the much fainter world beside the star. For example, this is how SPHERE captured the first-ever image of a multi-planet system orbiting another star like the sun in 2020.
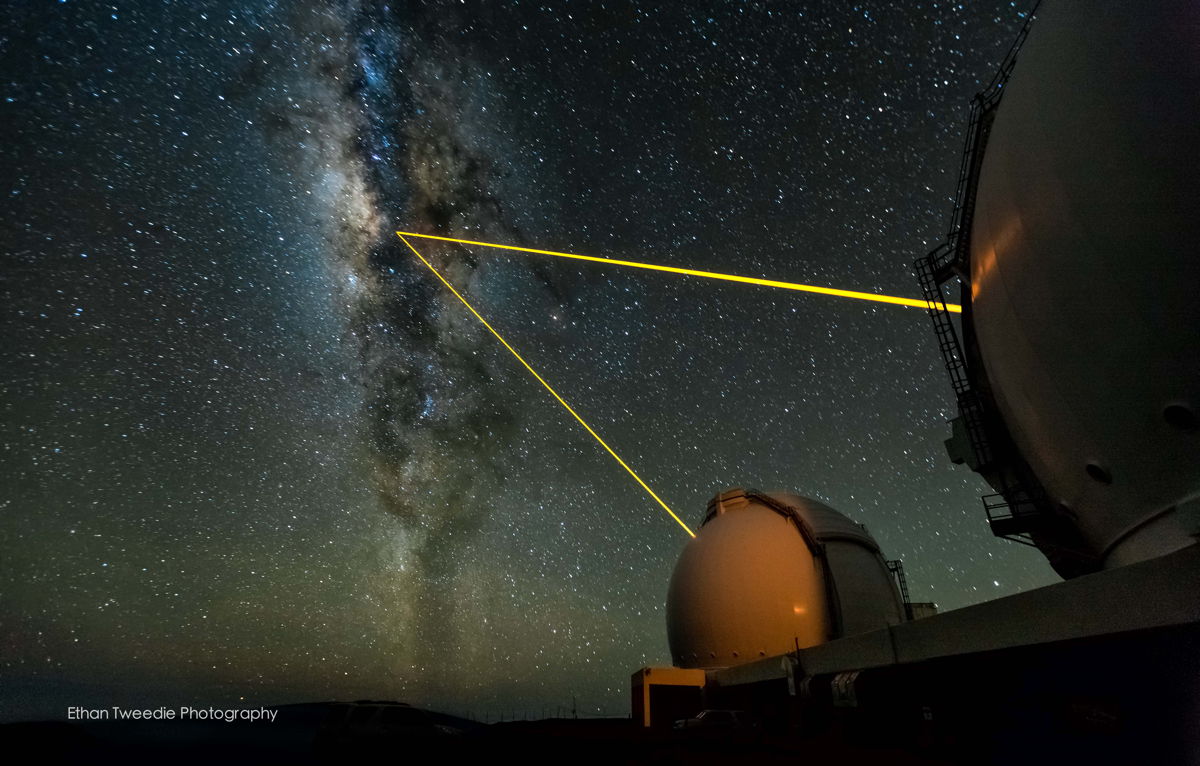
Although, at the moment, direct imaging is only possible for larger worlds that shine brightly in infrared light as a result from the heat they still contain from their birth. For example, the least massive exoplanet imaged with SPHERE is AF Leporis b, which is three times the mass of Jupiter.
It's a long way off imaging Earth-mass planets.
The aim, though, is to one day do that, and perhaps directly image an Earth-mass planet that also lies in the habitable zone of its star.
However, to be clear, it's not a given that the next generation of ground-based telescopes will be able to accomplish this. "Direct detection is really hard because you’ve got the extreme contrast ratio between the bright star and the very faint planets," said Verma. "Because of its large aperture, the ELT has the most chance of getting down to rocky planets and potentially something Earth-like over the TMT and GMT. Though it does require a level of correction for atmospheric disturbance with adaptive optics that is really challenging."
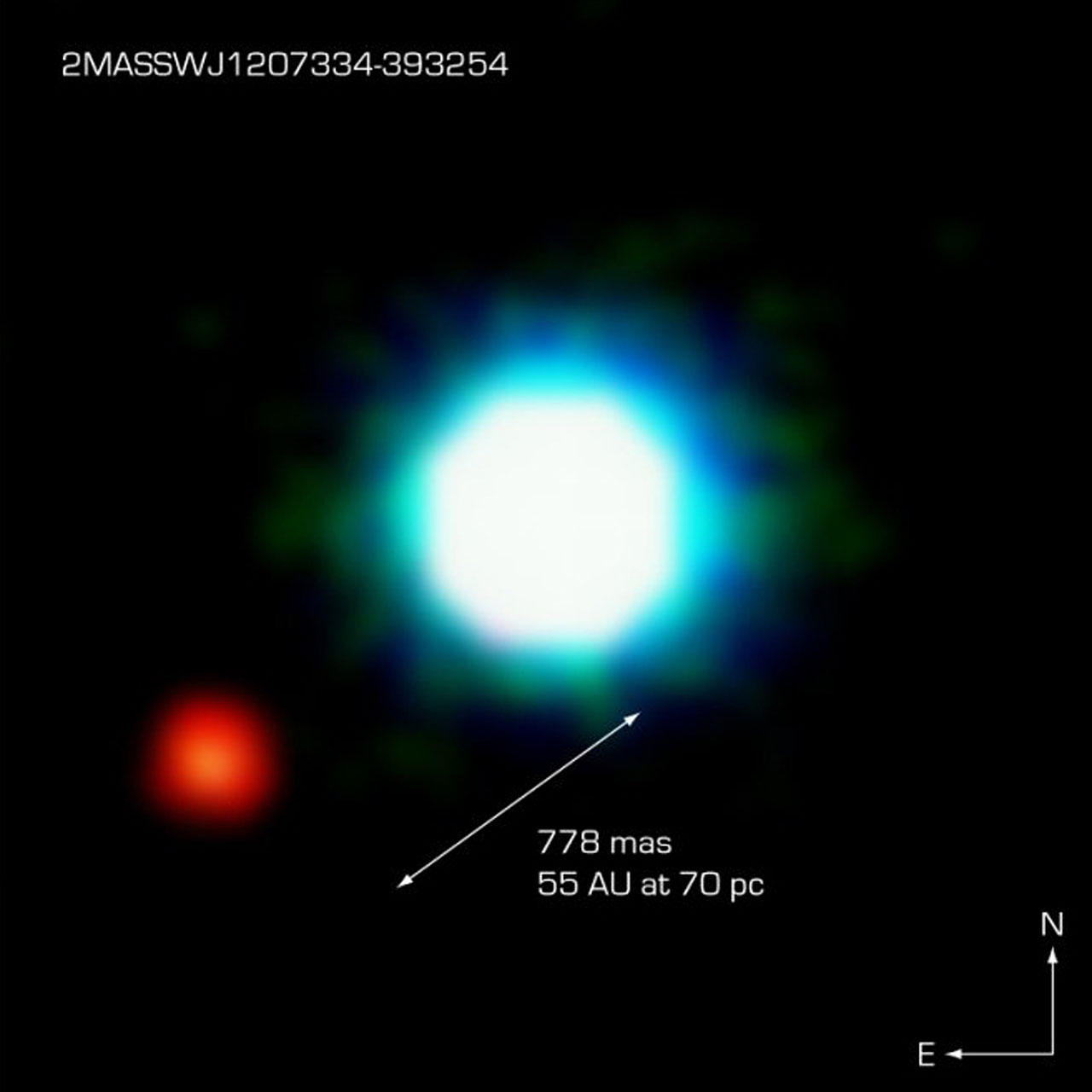
Adaptive Optics
Adaptive optics are one of the things that make these giant telescopes possible. Our planet's atmosphere is not conducive to observing the heavens clearly. Astronomers basically need to monitor how "still" the atmosphere is when observing the sky. We can see its deleterious effects in the way stars twinkle, as rising thermal currents create turbulence in the atmosphere. This enforces a limit on how well any telescope, no matter its size, can resolve a celestial object, with maximum resolution being about 0.5 arcseconds.
Because adaptive optics can get around this limitation, they really helped make telescopes in the 8 m to 10 m (315 inch to 394 inch) class possible in the 1990s; without adaptive optics, these large telescopes would have performed well below their capabilities. The idea of adaptive optics is for a telescope to focus on a guide star — either a real star that is bright enough, or an artificial star produced by firing a laser beam into the sky. A wavefront sensor is then able to read the light from this guide star, which would be scintillating all over the place as a result of turbulence, and computers can then control tiny actuators on a secondary or tertiary mirror, causing the mirror to flex on scales of milliseconds to counteract the turbulence and produce a clearer image of the guide star — and hence, whatever else the telescope might be looking at — with a far greater resolution.
This is the basics of how standard adaptive optics works, and it has allowed large ground-based telescopes such as Keck and the VLT to come closer to the capabilities of the spaceborne Hubble Telescope over the past 25 years. And this is certainly a fine way to image giant worlds far from their star. To directly image Earth-mass planets in their star's habitable zone, however, would require something even more special: extreme adaptive optics.
"Extreme adaptive optics has a higher-level order of correction for the atmospheric turbulence, and that allows you to reach the necessary contrast ratio [to see the faint planets next to their bright stars]," says Verma. "Ultimately, to find signs of life on Earth-mass planets we do need the combination of large apertures plus this extreme adaptive optics."
Building Bigger Telescopes
When astronomers led by Caltech's Mike Brown spotted a distant object in images taken by the 1.22-m (48-in) Samuel Oschin Schmidt telescope at Palomar, they knew they were onto something, and turned to the mighty Keck II telescope to confirm it. They'd found Eris, a dwarf planet more distant and more massive than Pluto, and about the same size (2,326 kilometers; 1,445 miles). Its discovery meant the end of Pluto as a fully fledged planet. Keck also discovered Eris' small moon, the 615-km (382-mile) wide Dysnomia, and worked with Hubble to measure Eris' mass as 27% more massive than Pluto.
Exactly how large telescopes can be made is a question of engineering, manufacturing, transport and gravity. Eight meters (315 inches) is about as large as a single telescopic mirror can be made from the spin-cast process pioneered by John Hill and J. Roger Angel. And even if single mirrors larger than this could be cast, it becomes physically difficult to haul them up a mountain. Gravity would also cause any larger single mirrors to sag and deform over time. Segmented mirrors, such as those used on the Keck and Gran Telescopio Canarias telescopes, are much easier to produce and transport, are not subject to sagging under their weight, and form a structurally more supported mirror.
Another development that has helped make the last quarter-century of telescopes possible is something called active optics. These shouldn't be confused with adaptive optics; rather than fixing blurring through atmospheric turbulence, active optics are designed to fix blurring as a result of mirror deformations or shaking.
As mentioned previously, the early large telescopes such as the Hale Telescope used chunky pieces of glass for their mirrors —so thick that they couldn't be easily deformed by the howling wind or the tremor of a minor earthquake. However, the more lightweight larger mirrors, whether spin-cast or segmented, are more susceptible to subtle deformations under vibrations. Even if the deformations are tiny, it would be enough to make the mirror lose focus. To compensate, computer-controlled actuators are added to the underside of a telescope's mirror and are able to adjust and reset the shape of that mirror so that it keeps focus whenever a gust of wind nudges the telescope. The system is referred to as active optics because the actuators are constantly operating, responding to minute changes in the mirror's shape in a matter of seconds.
What's threatening big telescope science?
Astronomers still don't know what dark matter is made from, but they are able to map its locations in the universe by looking at the way the gravity of this invisible substance is able to warp space and bend light, producing "gravitational lenses." In 2015, the Hyper Suprime-Cam on the Subaru Telescope began mapping dark matter by looking for where galaxy clusters featuring lots of dark matter were creating gravitational lenses. The intent was not only to map dark matter, but to understand how its distribution changes in different epochs in cosmic history to, in turn, see how dark energy's impact on the expansion of the universe is changing the distribution of this strange substance.
The science being conducted on these large telescopes isn't just confined to exoplanets. The light-gathering power of their large apertures allows them to be used to probe the early universe, particularly spectroscopically, learning things like how galaxies grew during the first few billion years of cosmic history. Closer to home, they can also study star formation in nearby galaxies, or the effects of feedback from active black holes in galaxies on the stars and interstellar gas around them. Large telescopes are used to chase down the afterglows of gamma-ray bursts and kilonovas, and to study how dark energy is affecting the expansion of the universe.
However, future astronomical research in these areas is in danger.
Research in 2022 by scientists at the University of Bern and the National Centre of Competence in Research (NCCR) Planets has shown that the locations where many of our largest optical telescopes are – the Canary Islands, Chile, Hawaii and so on — will experience an increase in temperature and water content (and hence cloud coverage) in the atmosphere by the middle of this century as the global climate warms primarily due to human activities. This will reduce the number of clear nights, increase the risk of condensation on a telescope's mirror at night and increase the air turbulence within and immediately around the telescope's dome, never mind high up in the sky, where adaptive optics will need to work overtime.
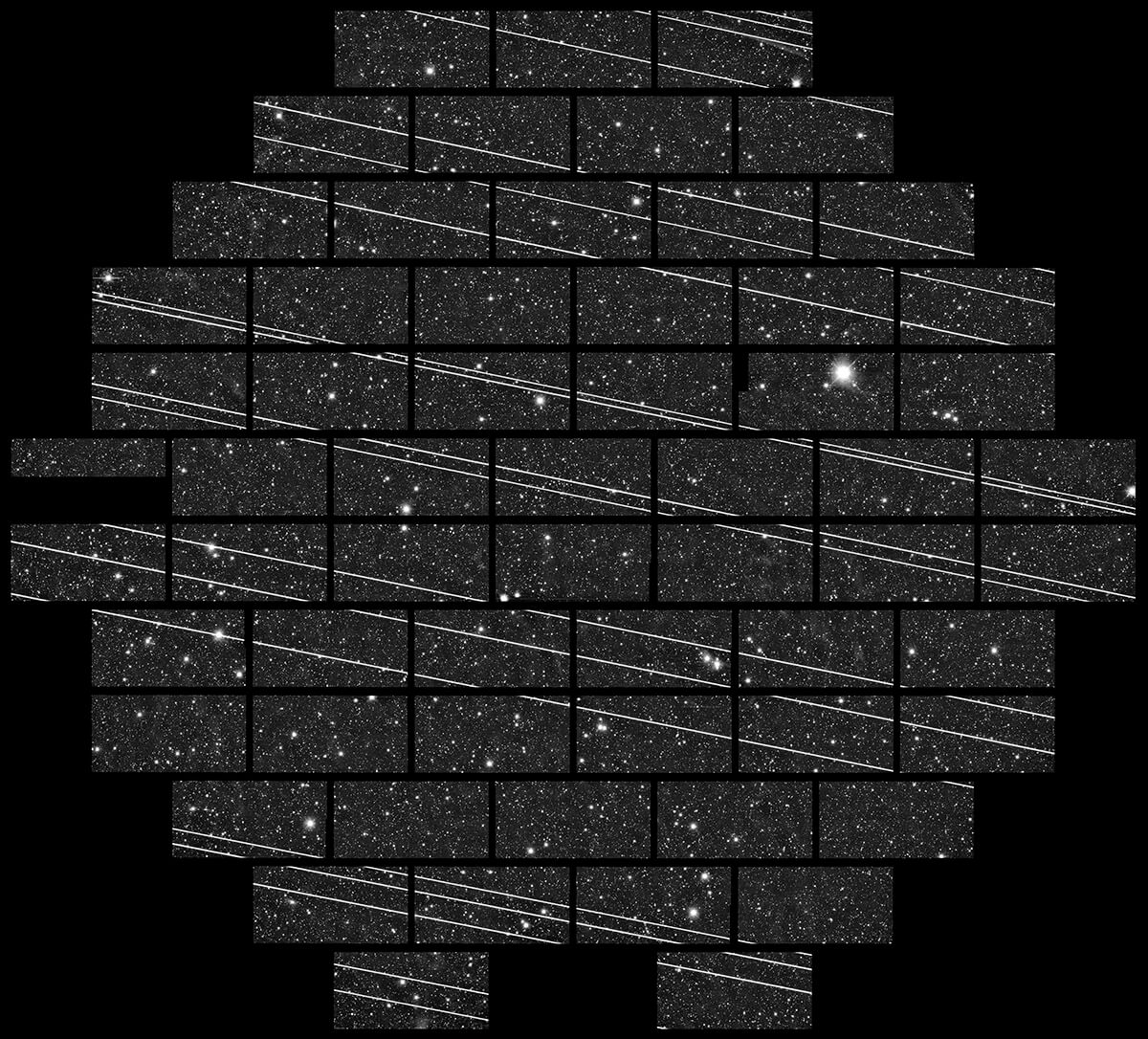
In 2010 astronomers using the VLT were able to spectroscopically analyze the atmosphere of a super-Earth for the first time. Although the planet, GJ 1214b, is too close to its star to be in the habitable zone, the VLT was able to narrow down the options for the atmosphere to either have a steamy, water-rich atmosphere, or be covered by hazy clouds like Venus. The planet itself has a mass 6.5 times that of Earth and orbits a star 40 light-years from us.
But if the changing climate isn't enough to stymy the work of our large observatories, satellite constellations could potentially be the final nail in their coffin. There are currently around 10,000 active satellites in space, many launched in recent years as part of satellite constellations such as Starlink, and tens of thousands more are set to be launched. Already, these satellites are interfering with astronomical observations, their trails ruining images as they pass silently through a telescope's field of view. This really hit home around the beginning of this decade, when images from telescopes such as the Victor M. Blanco 4-meter telescope at the Cerro Tololo Inter-American Observatory in Chile revealed dozens of satellite trails clogging up even short exposures taken through the telescope. Software can remove these trails up to a point, but they are a major headache for astronomers.
However, in most cases, the larger your telescope, the less of a problem satellites are. That's because as your aperture increases, the size of your field of view usually decreases. This does make sense — you build larger telescopes to see smaller objects, or smaller details in objects. It's like a magnified view that covers a smaller area of sky. The exception is the Vera C. Rubin Observatory in Chile, which has an 8.4-m (331-in) telescope designed to have a wide field (3.5 degree) field of view for surveying the entire night sky every few days in detail, looking for asteroids, supernovas, active black holes and other transients.
Estimates have suggested that, when it begins astronomical observations in a few years' time, up to 50% of its observations could be affected by satellite constellations.
"If the satellite networks stay as they are now, I don't think it would be a huge problem for the ELT, because as the field of view gets smaller, the impact of satellites is less," said Verma. "But if things keep going the way they are, the future of ground-based astronomy will be called into question. It could be that our only option will be to do astronomy from space."
Should that happen, the era of large-aperture, ground-based mega-telescopes that has dominated the past 25 years of optical astronomy will be effectively over. While best results are still obtained from space, the James Webb Space Telescope cost almost $10 billion, whereas the ELT will cost about $1.6 billion. Astronomy from space is expensive, so space telescopes will be few in number, leaving many astronomers around the world without easy access to a large telescope.
It almost doesn't bear thinking about. The past 25 years have been a golden age for optical astronomy, as chronicled in the stories presented on Space.com over that time period. Hopefully, when we celebrate our 50th anniversary, we won’t be talking about the death of ground-based astronomy, but of all the amazing new discoveries that it has made.