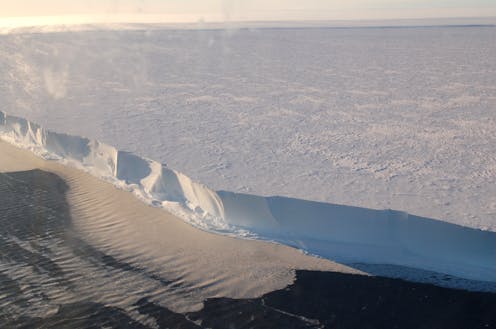
The size of the Antarctic ice sheet can be hard to comprehend. Two kilometres thick on average and covering nearly twice the area of Australia, the ice sheet holds enough freshwater to raise global sea levels by 58 metres.
Ice loss from this sheet is projected to be the leading driver of sea level rise by 2100, yet its contribution remains highly uncertain. While sea levels are certain to rise this century, projections of the contribution from Antarctic ice vary from a 44 cm rise to a 22 cm fall.
Much of this uncertainty is because the ocean processes that control the fate of the sheet occur on an incredibly small scale and are very difficult to measure and model.
But recently scientists have made significant progress in understanding this “ice-ocean boundary layer”. This progress is the subject of our new review paper, published today in Annual Reviews.
Shrinking, thinning and retreating
At the margins of the Antarctic ice sheet, glaciers flow into the Southern Ocean, forming floating ice shelves. These ice shelves act as keystones, stabilising the ice sheet. They’re also shrinking.
The ocean melts ice shelves from below – a process known as “basal melting”. Increased basal melting has led to the thinning and retreat of the ice sheet in some regions, raising global sea levels.
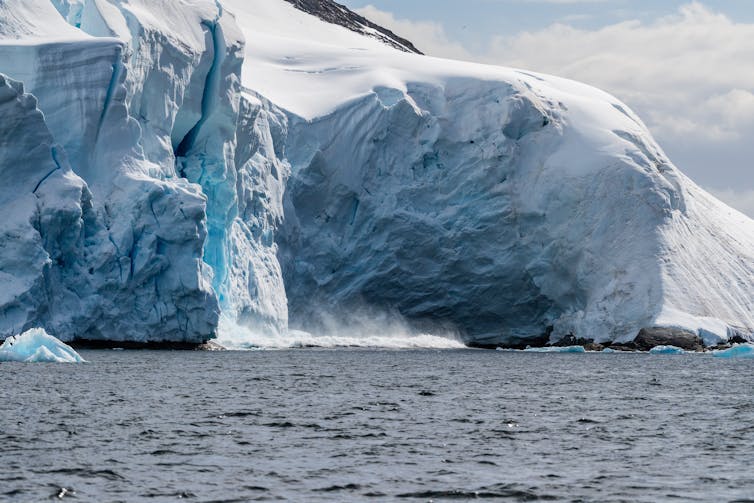
It has also slowed the deepest current in the global overturning circulation, a system of ocean currents that circulates water around the globe.
Like the glaciers that feed them, ice shelves are immense. Yet the ocean processes that control basal melting, and the fate of the entire Antarctic ice sheet, occur on the scale of millimetres. They happen in a thin layer of ocean, just beneath the ice.
The boundary layer between the ice shelf and the ocean is cold, miles from anywhere, and beneath very thick ice, so it’s no wonder it has hardly been measured at all.
Studying this layer with other techniques such as computer simulations is also a huge challenge. Until recently, the tiny motions within the ice-ocean boundary layer put accurate modelling of ice melt out of reach.
These twin challenges have long stymied efforts to answer the deceptively simple question: “How does the ocean melt Antarctic ice shelves?”
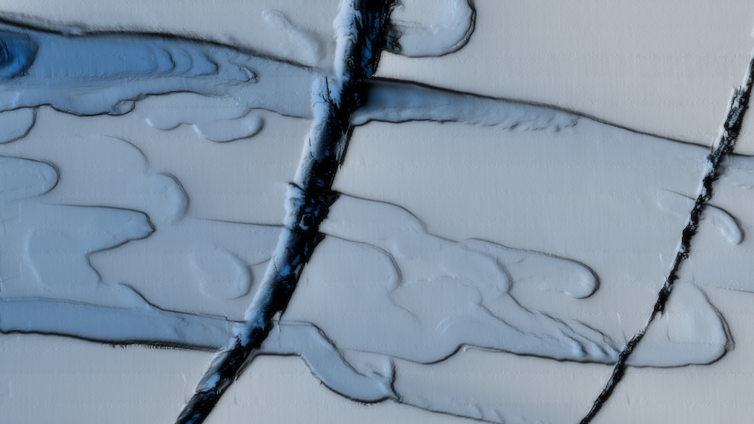
Modelling the micro-scale
Computer simulations of ocean processes aren’t new.
But only recently have simulations of the ice-ocean boundary layer become feasible, as computing resources grow and the cost of using them shrinks.
Several research groups around the world have taken on this problem, modelling the micro-scale ocean flow that supplies heat to the ice for melting.
Researchers are looking for a relationship between what the ocean is doing, and how quickly the ice melts. So far, they’ve uncovered not just one relationship but several, each indicating a different melt “regime”. Ocean conditions (temperature, salt content and the speed of ocean currents) and the shape of the ice determine which melting regime applies.
Ice sheet shape is key because meltwater is fresh and lighter than the surrounding ocean. Like hot air collecting at the top of a room, fresh, cold meltwater collects in hollows in the lower surface of the ice sheet, insulating the ice from the ocean water below and slowing melting.
For steeply sloping ice, the insulating effect is much less. The energetic flow of meltwater as it rises under steep ice leads to mixing with the warmer ocean waters. This increases melting.
Fast ocean currents have a similar effect, as they transfer heat to the ice.
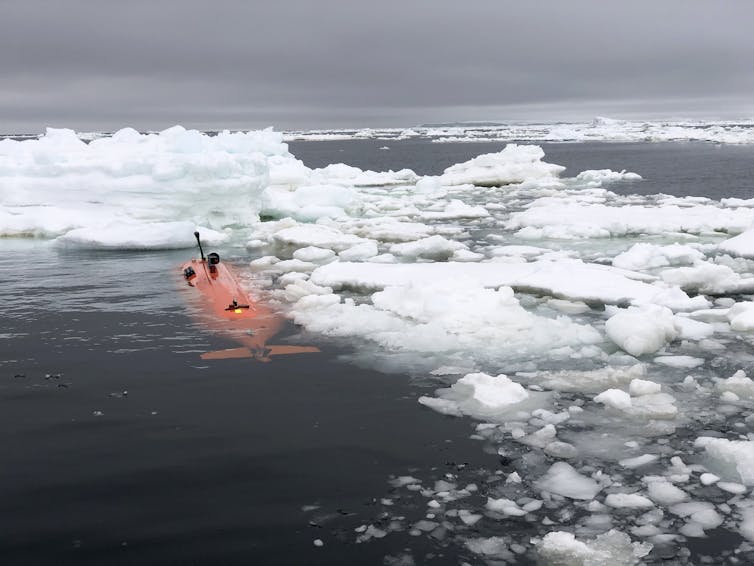
Sonar-fitted robots
Recently, ocean robots, including autonomous underwater vehicles and tethered probes deployed by drilling through the ice, have provided unprecedented amounts of data on the environment beneath ice shelves.
Using sonar and cameras, these robots have revealed a weird and wonderful “icescape” on the underside of ice shelves.
This icescape is made of many different ice features, ranging from centimetres to kilometres in size. Some, like steep-sided crevasses, are formed by ice fracturing. Others, like dimpled depressions in the ice (often called “scallops”), stair-like “terraces”, mussel-shaped “scoops”, and larger basal channels, are thought to be formed by melt processes.
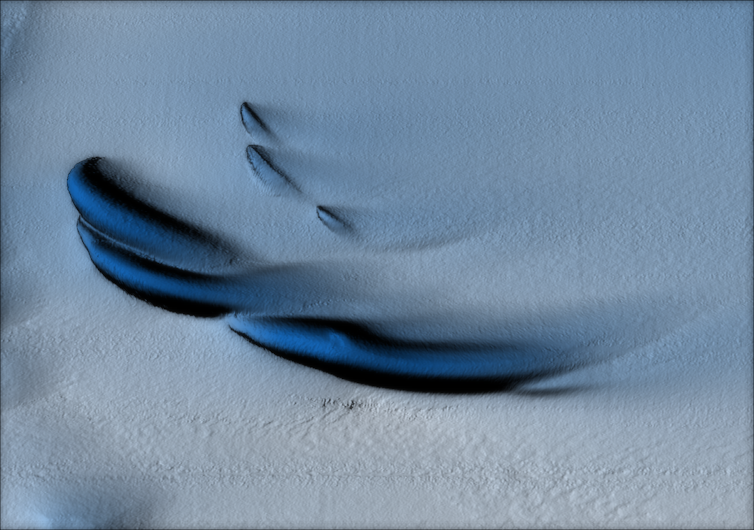
Our new knowledge of melting from computer simulations and robots sheds light on these features and how they form. The existence of melt regimes helps explain the evolution of steep-sided terraces, or why different features appear in distinct parts of an ice shelf.
For instance, in the warm, calm eastern part of the Dotson ice shelf in west Antarctica, an autonomous robot observed basal terraces. In the west of Dotson – which experiences cold, fast currents – large mussel-shaped scoops were discovered.
Uncertainties remain
Exactly how some of these features form is still unknown.
New simulations that allow the ice-water boundary to move in time show the “self-sculpting” behaviour of ice melt. This is similar to how dunes form and move in a desert.
However, new computer models are needed to simulate the formation and evolution of the whole icescape.
Some of the recent advances highlighted here are helping to reduce the uncertainty in our understanding of the contribution of the Antarctic ice sheet to global sea level rise.
However, incorporating our new understanding of basal melt, and the dynamic icescape it forms, into climate and ice sheet models still presents a huge challenge.
Overcoming this challenge is urgent. Accurate representation of melt in climate and ice sheet models will reduce the deep uncertainty in sea level rise projections, especially as ocean conditions – and ice shelf melt regimes – shift into the future.

Madelaine Gamble Rosevear receives funding from the Australian Research Council.
Ben Galton-Fenzi is employed by the Australian Antarctic DIvision.
Bishakhdatta Gayen is employed by the University of Melbourne. He receives funding from the Australian Research Council. He is affiliated with Indian Institute of Sciences.
Catherine Vreugdenhil receives funding from the Australian Research Council.
This article was originally published on The Conversation. Read the original article.