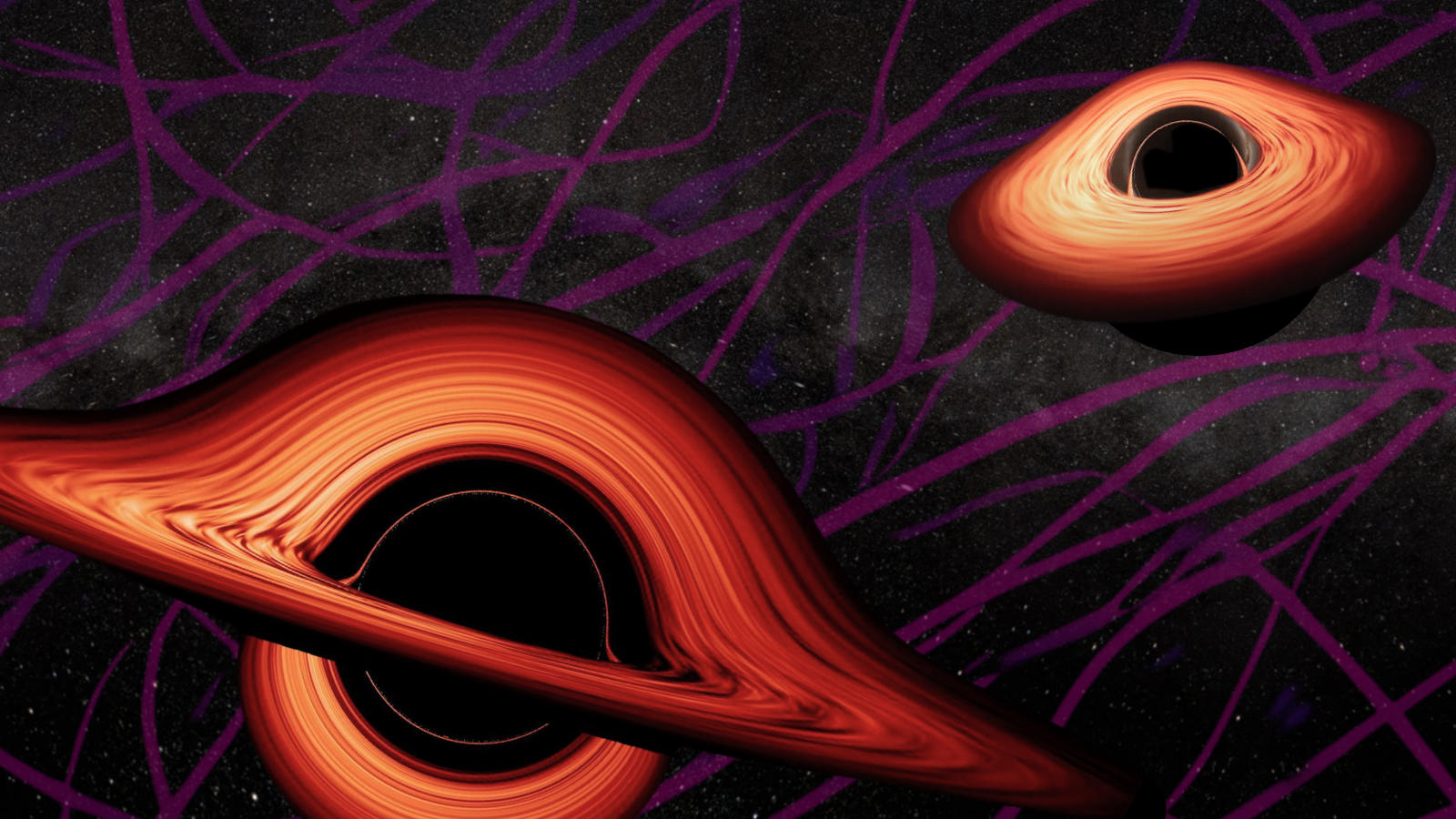
Dark matter, the most mysterious "stuff" in the universe, may be a sort of cosmic matchmaker, allowing supermassive black holes to overcome a final hurdle before colliding and merging into one.
Astronomers have understood for some time that when galaxies clash to create larger, misshapen galaxies, the supermassive black holes that sit at their centers draw together as well. Eventually, these black holes also merge, creating an even more monstrous supermassive black hole and a high-pitched "scream" of ripples in the very fabric of space and time called gravitational waves.
While the detection of gravitational waves has provided us with evidence of this collision process, and has become indeed fundamental for our understanding of how supermassive black holes obtain masses equivalent to millions or even billions of suns, there's a lingering problem.
Astrophysicists have good models of the later stages of supermassive black hole mergers, but scientists still don't fully understand how these cosmic titans cross the final approximately 3.3 light-years (also known as a "parsec") between them to trigger the merger process. This issue has become known as the "final parsec problem."
New research suggests the solution could come from the dark universe.
Related: Heaviest pair of black holes ever seen weighs 28 billion times more than the sun
Dark matter could help supermassive black holes "break the ice" and begin the coupling process — but there's a catch (isn't there always?). This theory only works if dark matter particles interact with each other.
"The process by which supermassive black holes merge is complex because it relies on the black holes finding each other, forming a binary system, and then the orbital separation between them slowly decreasing," research team leader Gonzalo Alonso-Álvarez from the University of Toronto told Space.com. "When the separation between the two black holes is around a parsec or so, scientists were worried because, due to existing calculations, it looked like the merger would stall.
"We suggest the presence of dark matter, which is thought to be very dense at the center of the galaxies, can have a significant effect and help those black holes merge."
Alonso-Álvarez explains that the exciting thing about this finding, based on mathematical modeling, is that it hinges on dark matter self-interactions. More specifically, to "play matchmaker" between black holes, dark matter particles must scatter off each other upon collision.
For that reason, following this theory could not only resolve the final parsec problem, but it might also finally reveal what dark matter is composed of, unraveling one of the most fundamental mysteries in science.
A dark matter diversion
Before discussing the role dark matter could play in facilitating black hole mergers, it is worth a little diversion to explain why this mysterious cosmic substance poses such a troubling problem for scientists.
Dark matter doesn't interact with light — or, if it does, the interaction is so weak we can't detect it. This makes it effectively invisible. And, because electrons, protons and neutrons — particles that make up the atoms in everyday matter — do interact with light, scientists know dark matter can't be made up of these particles.
This has spurred a massive search for particles outside the Standard Model of particle physics.
But that isn't the extent of the dark matter problem. Not only is this substance possibly composed of particles that don't fit into the best model of the subatomic world that we have, but they also absolutely dominate "everyday" matter particles in terms of mass.
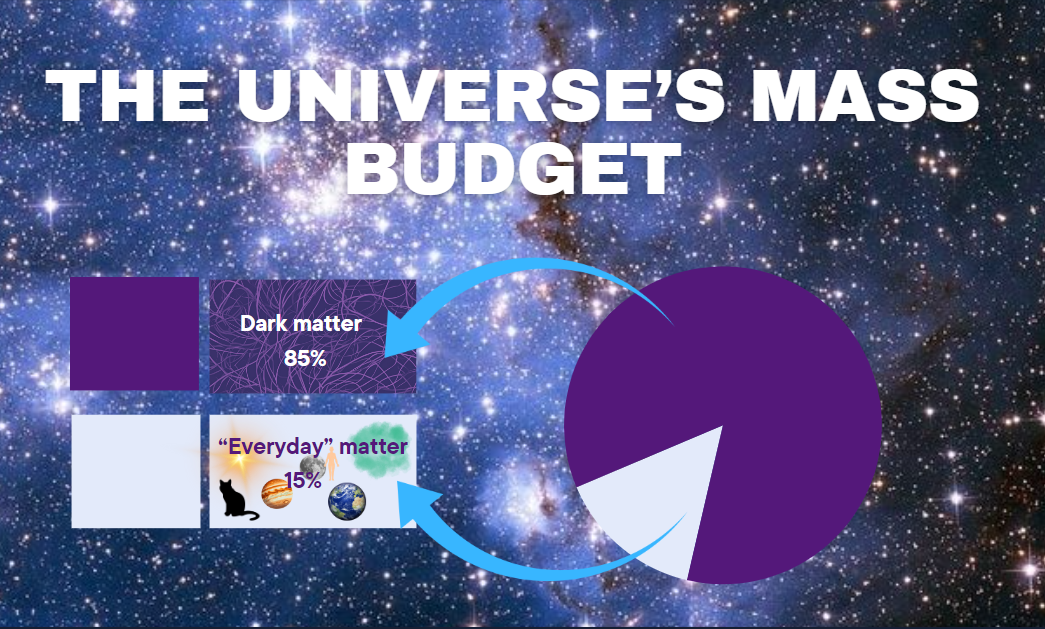
Dark matter particles "outweigh" ordinary particles by a ratio of between 5 to 1 and 6 to 1. That means all the "stuff" you see around you — stars, planets, moons, vast gas clouds in space, other humans, your coffee table, monitor, mouse and even next door's cat — account for just 15% of mass in the cosmos.
That leaves a lot of stuff in the universe we can't explain.
We know dark matter exists because despite not interacting with light or ordinary matter, dark matter does interact with gravity. When it does, the effects it causes can influence the behavior of ordinary matter and light. For instance, if it weren't for the gravitational influence of dark matter, researchers have found that gravity generated by the mass of the stars, gas, planets and moons in galaxies wouldn't be enough to prevent them from flying apart as they zoom around.
Cosmic Tinder
Alonso-Álvarez explained that, when galaxies first merge, their respective supermassive black holes are far apart. Eventually, they begin their respective journeys toward the center of those merging galaxies, which will become the site of their own merger and the heart of a newly created conglomerate galaxy.
This journey is facilitated by the black holes' loss of energy, and it happens in three distinct stages.
During "stage one," the black holes sweep past a multitude of stars and engage in gravitational interactions with those stars. Due to those interactions, the black holes pass along velocity and slow down. This loss of velocity allows the concentration of mass at the center of the merger to gravitationally influence the black holes to head (somewhat leisurely) toward one another.
That loss of energy continues to be important after the final 3.3 light-years are crossed and the supermassive black holes form a binary system, which is stage three of this process. At this point, the voids swirl around each other, generating gravitational waves. As these waves ripple away from the binary system, they carry with them angular momentum, thus losing rotational kinetic energy.
This very efficient loss of energy causes the two black holes to draw closer together, with their merger becoming unstoppable. The tighter their orbits become, the faster gravitational waves are radiated away. Eventually, as the supermassive black holes get closer and closer together, their gravitational influence takes over, and they collide.
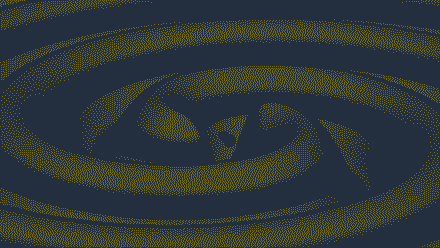
There is a problem, however, and one you may have clocked. What happens in stage two? That's the step between losing energy to stars and losing energy via gravitational waves.
"The interactions with stars are really only effective when the black holes are very far apart from each other, so distances larger than a parsec, and gravitational wave emission only really kicks in when they are closer than a fraction of a parsec," Alonso-Álvarez said. "There was this separation of around the parsec, where nothing was really working in previous models to deliver a real mechanism by which the orbit could keep shrinking."
That's where dark matter might come in.
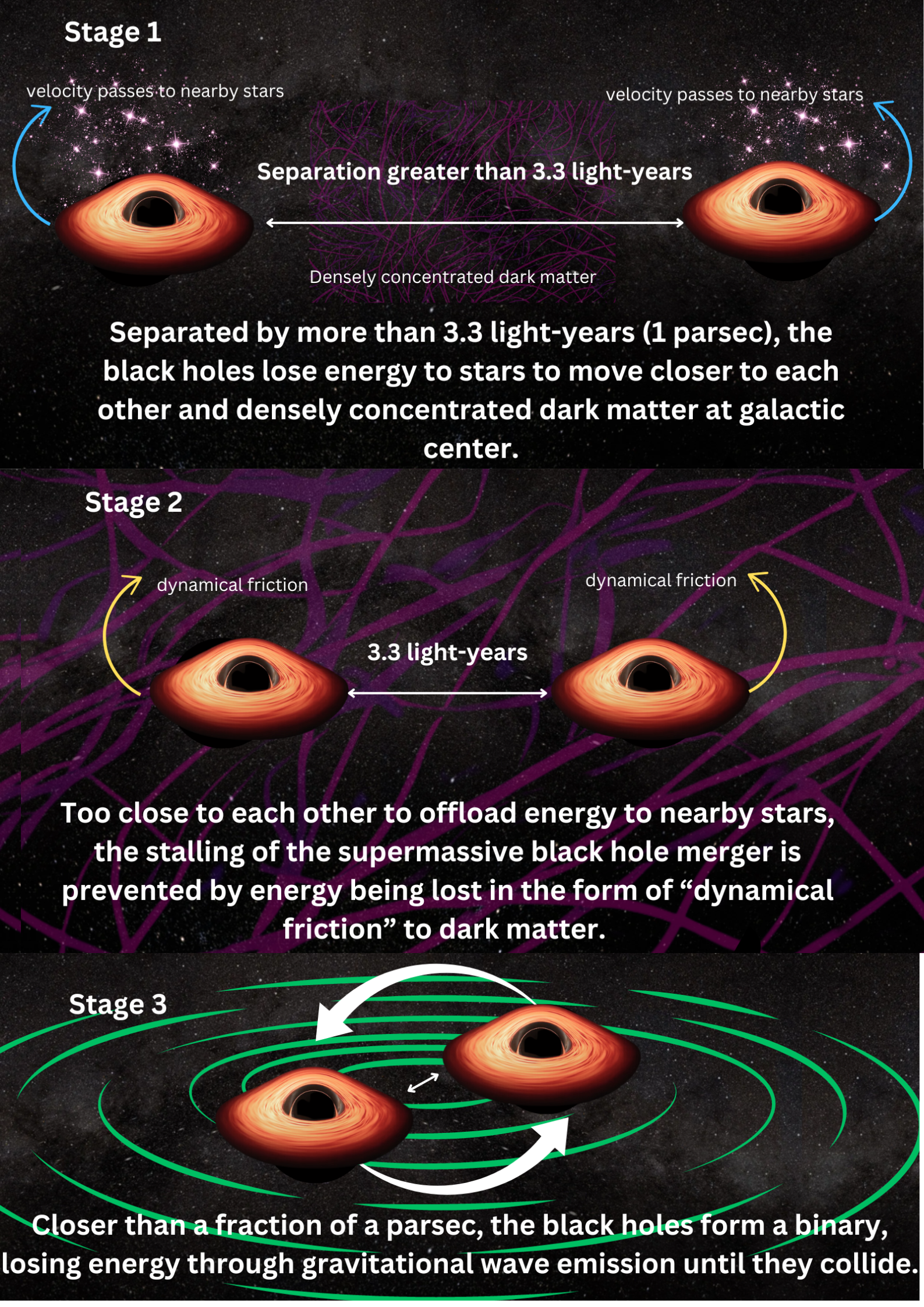
Alonso-Álvarez and colleagues suggest that when the supermassive black holes reach a separation of 3.3 light-years and can no longer offload energy to stars, dark matter takes over and starts stripping energy away through a process called "dynamical friction."
"If the black holes are moving in this dense 'dark matter fluid,' then they generate friction like an object moving through a viscous liquid," Alonso-Álvarez continued. He added that if dark matter is modeled as a fluid, interactions between dark matter particles grant it a high viscosity. A high viscosity for a normal liquid indicates how difficult it is for an object to pass through. Water has a low viscosity compared to molasses, for example.
"In this case, this friction is generated only by gravity," Alonso-Álvarez said. "It never interacts with the black hole via a force other than gravity."
Furthermore, if this theory is correct, then it also tells us something about dark matter.
Scatter, dark matter!
Not all models of dark matter suggest particles of this mysterious substance can scatter off each other.
"Without dark matter scattering, this mechanism just would not work," Alonso-Álvarez said. "The reason for this is the energy that the black holes are losing to the friction, and the dark matter goes into increasing the velocity of the dark matter particles. If there's no viscosity, the dark matter particles would be dispersed away from this final parsec region."
That would mean, after a short time, there would be no more dark matter in this central region that could generate the necessary friction.
"However, if there are dark matter self-interactions, what happens is this energy is redistributed between the particles in the central region of the galaxy so that the dark matter is not dispersed away," Alonso-Álvarez added. "This is crucial."
The interaction between dark matter and supermassive black holes could also explain calculations of dark matter haloes around galaxies that have shapes that can't fully be explained by "cold dark matter" models. These cold dark matter models suggest this mysterious stuff is slow-moving (hence "cold") and does not self-interact. If Alonso-Álvarez and colleagues' dark matter matchmaker theory is accurate, it may, therefore, necessitate a move away from cold dark matter.
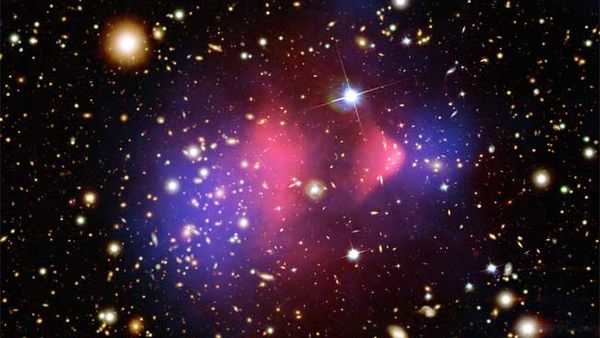
Additionally, to facilitate the interaction between dark matter particles, there must be an unknown "force-carrier" particle, and this research gave the team some clues as to the nature of this particle. A force-carrying particle, or "boson," acts as a go-between, helping other particles interact. For example, the electromagnetic force uses photons, or particles of light, as its force carrier, while gravity is hypothesized to use a boson called a gluon.
"We started off very naively thinking that the dark matter self-interactions should just help bridge this final parsec problem, but we didn't think we would be able to predict what kind of self-interactions are favored," Alonso-Álvarez continued. "As we were working and kept refining our calculations, we found that a very particular model where the force carrier has a mass which is in the order of 1% of the mass of the dark matter particle that is really preferred and no other thing that we tried worked."
"The calculations that we did for this paper were purely analytical. We're currently working on developing simulations to understand this mechanism a bit better," Alonso-Álvarez said.
The physicist added that observational evidence for this theory could be provided by collections of highly precise rotating neutron stars called pulsar timing arrays, which scientists use as accurate cosmic time-pieces. Critical to this will be the study of the background "hum" of the cosmos, called the "gravitational wave background," which originates from supermassive black hole mergers across the history of the universe.
"One can look at the impact of this dark matter and friction on that signal and make a prediction about how the presence of dark matter modifies the signal," Alonso-Álvarez added. "I think the most interesting thing is one could at the same time correlate this with the evidence for the presence of dark matter self-interactions at galactic scales of many thousands of parsecs."
Alonso-Álvarez and the team's findings are discussed in a paper published in the journal on July 9 in the journal Physical Review Letters.