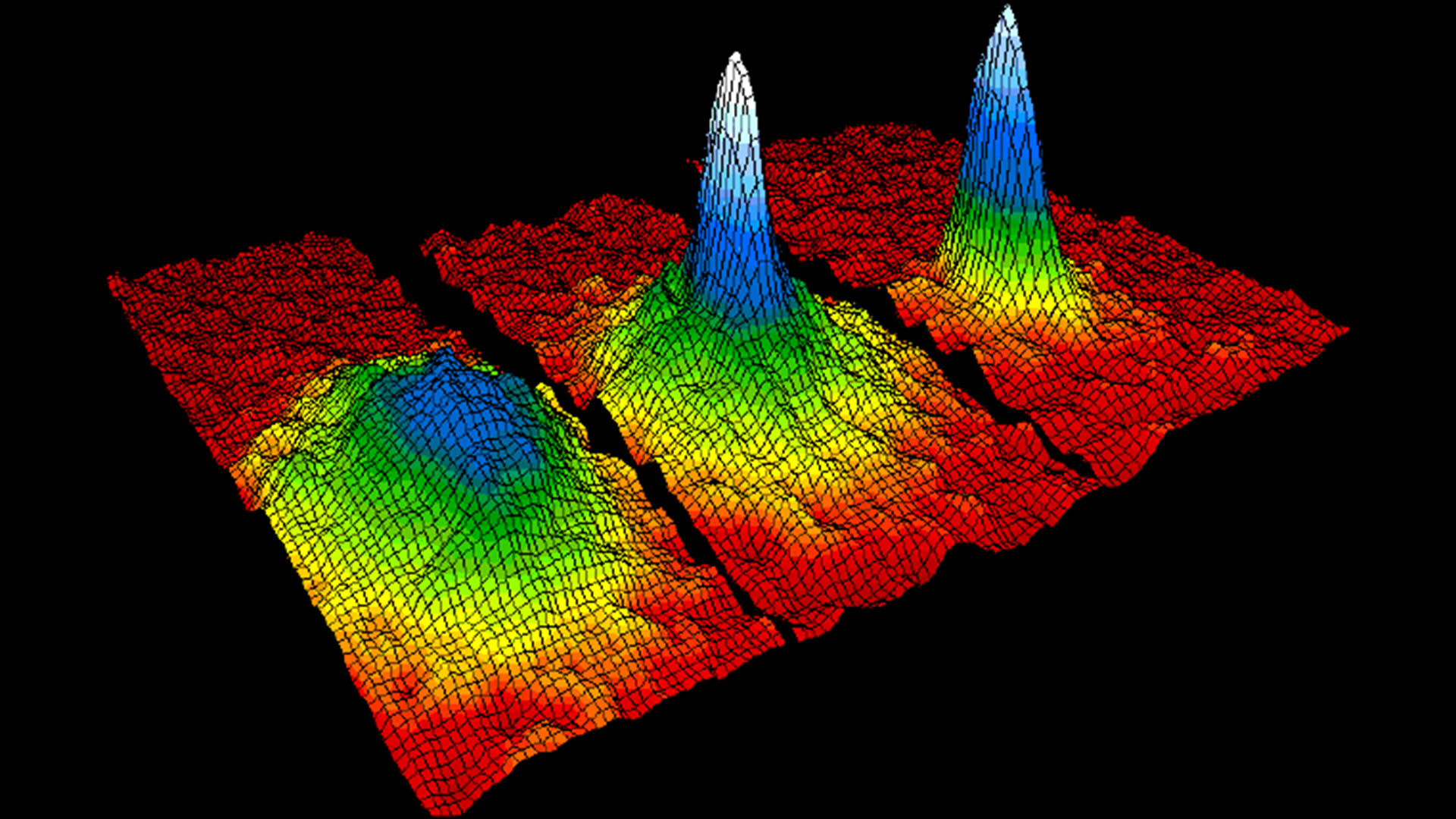
The Bose-Einstein condensate (BEC) is one of the five primary states of matter. In it, atoms reach such low energies that the rules of quantum mechanics dictate that they stop acting as individual atoms and behave like a single "super atom."
A Bose-Einstein condensate forms only when materials are cooled to within a hair of absolute zero. At that temperature the atoms are hardly moving relative to each other; they have almost no free energy to do so. The atoms then begin to clump together, and enter the same energy states. They become identical, from a physical point of view, and the whole group starts behaving as though it were a single atom.
Gases, liquids, solids and plasmas have been studied for decades, if not centuries, but Bose-Einstein condensates weren't created in the laboratory until the 1990s. To make a Bose-Einstein condensate, you start with a cloud of diffuse gas. Many experiments start with atoms of rubidium. Then you cool it with lasers, using the beams to take energy away from the atoms. After that, to cool them further, scientists use evaporative cooling.
Related: Inside the 20-year quest to unravel the bizarre realm of 'quantum superchemistry'
"With a [Bose-Einstein condensate], you start from a disordered state, where kinetic energy is greater than potential energy," Xuedong Hu, a professor of physics at the University at Buffalo, told Live Science. "You cool it down, but it doesn't form a lattice like a solid."
Instead, the atoms fall into the same quantum states, and can't be distinguished from one another. At that point the atoms start obeying what are called Bose-Einstein statistics, which are usually applied to particles you can't tell apart, such as photons, or light packets.
Theory and discovery
Bose-Einstein condensates were first predicted theoretically in the 1920s by Satyendra Nath Bose (1894-1974), an Indian physicist who also discovered the subatomic particle named for him, the boson. Bose was working on statistical problems in quantum mechanics, and sent his ideas, which pertained to photons, to Albert Einstein according to the American Physical Society.
Einstein thought them important enough to get them published. As importantly, Einstein saw that Bose's mathematics — later known as Bose-Einstein statistics — could be applied to atoms as well as light. The two published a series of papers laying out the details of this strange form of matter in 1924, according to the APS.
What the two found was that ordinarily, atoms have to have certain energies — in fact one of the fundamentals of quantum mechanics is that the energy of an atom or other subatomic particle can't be arbitrary. This is why electrons, for example, have discrete "orbitals" that they have to occupy, and why they give off photons of specific wavelengths when they drop from one orbital, or energy level, to another. But cool the atoms to within billionths of a degree of absolute zero and some atoms begin to fall into the same energy level, becoming indistinguishable. That's why the atoms in a Bose-Einstein condensate behave like "super atoms." When one tries to measure where they are, instead of seeing discrete atoms one sees more of a fuzzy ball.
Other states of matter all follow the Pauli Exclusion Principle, named for physicist Wolfgang Pauli. Pauli (1900-1958) was an Austrian-born Swiss and American theoretical physicist and one of the pioneers of quantum physics. His principle dictates that fermions — particles such as quarks and leptons that make up matter — can't be in identical quantum states. This is why when two electrons are in the same orbital, their spins must be opposite so they add up to zero. That, in turn, is one reason why chemistry works the way it does and why atoms cannot occupy the same space at the same time. Bose-Einstein condensates break that rule.
Though Bose and Einstein's work predicted such states of matter should exist, it wasn't until 1995 that a team led by Eric A. Cornell and Carl E. Wieman, both of the Joint Institute for Lab Astrophysics (JILA) in Boulder, Colorado, managed to make one of rubidium atoms, and MIT's Wolfgang Ketterle and colleagues, made one of sodium atoms, that we had experimental confirmation of their existence. The trio shared the 2001 Nobel Prize in Physics for this work.
In July 2018, an experiment aboard the International Space Station cooled a cloud of rubidium atoms to ten-millionth of a degree above absolute zero, producing a Bose-Einstein condensate in space. They repeated the demonstration of a BEC on the ISS in 2020.The experiment also now holds the record for the coldest object we know of in space, though it isn't yet the coldest thing humanity has ever created.
And in 2023, scientists at the University of Chicago created the first BEC which demonstrated a strange phenomenon, called quantum superchemistry. In that strange quantum phenomenon, individual atoms in a BEC chemically react all at once. In the experiment, scientists convincingly showed that thousands of cesium atoms bonded all at once to form cesium molecules, then near-instantaneously converted back to cesium atoms.