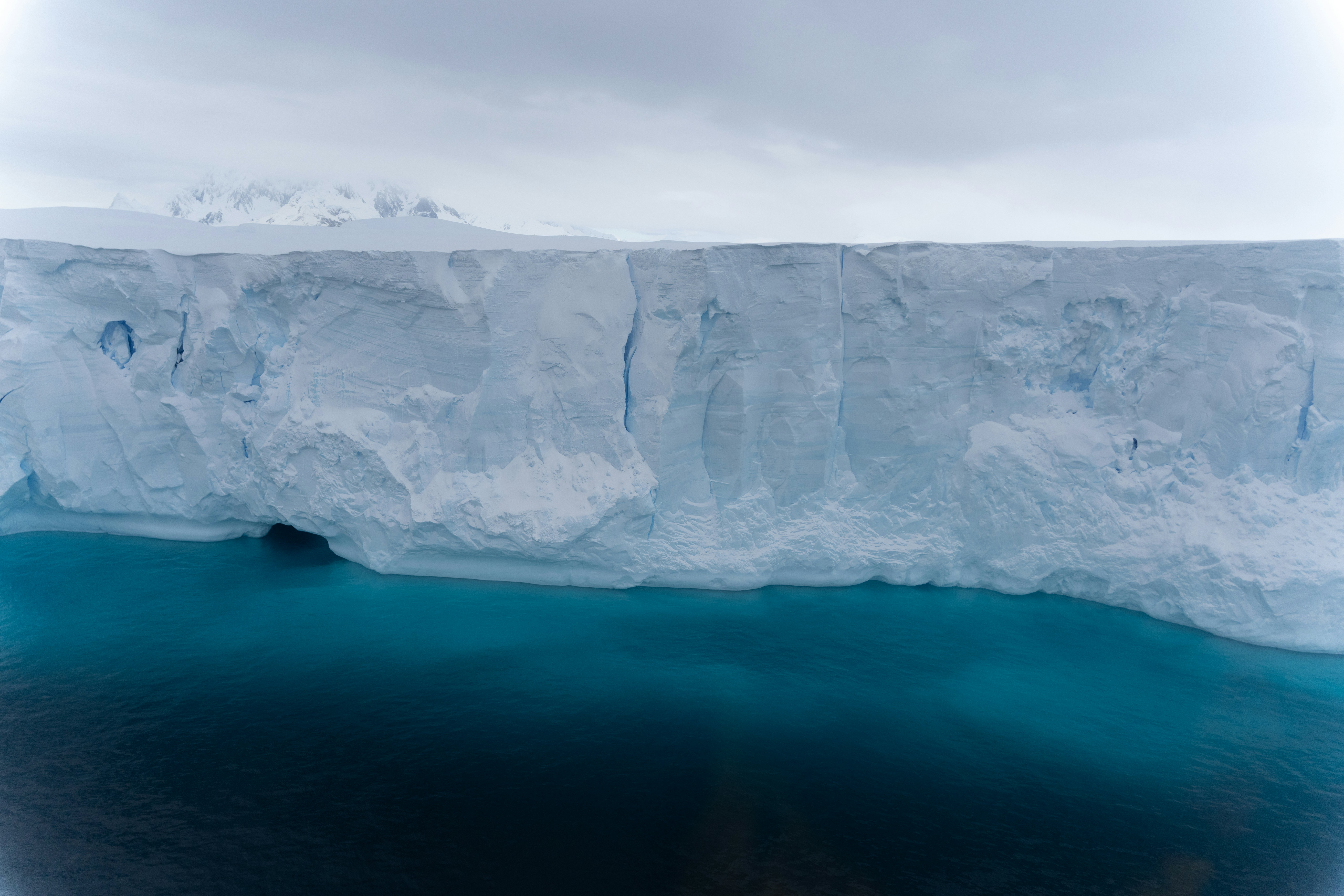
Residents of flood-prone cities such as Miami, London, and New York live with the fear of rising waters inundating their homes, transit systems, and businesses during storms. As the waters creep higher each year, people wonder: How far — and how fast — will sea level rise in the years to come? The answer, in large part, lies in the stability of the vast ice sheets in Greenland and Antarctica, including the Thwaites Glacier in Western Antarctica, the so-called Doomsday Glacier.
As an ever-growing mountain of research confirms that climate change is leading to more rapid melting of polar ice, the race is on to determine when, where, and how polar ice sheets will collapse. This collapse could raise sea level by 6 feet or more by the end of the century — or even sooner.
Glaciologist Richard Alley of Penn State University has studied polar ice for more than 35 years. In the 1990s, his ice-core research shocked the world with the startling finding that the last Ice Age did not come to a gradual end, as long believed, but ended abruptly and violently, with temperatures rising sharply in three short years.
These days, Alley focuses his attention on ice shelves, the extensions of polar glaciers into the sea. Here, massive ice cliffs break off into the ocean in a process called calving. Researchers are still working to understand why some ice shelves calve faster than others and what triggers this instability. Alley and his colleagues explore current research on calving in the 2023 Annual Review of Earth and Planetary Sciences.
Knowable Magazine spoke with Alley about his work on the calving of ice sheets and about coping with the ice’s uncertain future. This conversation has been edited for length and clarity.
Why study ice shelves?
Greenland and Antarctica are covered in humungous piles of ice. All piles tend to spread under their own weight like a ladleful of pancake batter spreads in the pan. If your ice pile spreads into a cold ocean under cold air, it forms floating extensions called ice shelves. These shelves are almost all the way around Antarctica, and there are still a few left in Greenland.
Ice shelves generally have slower-moving ice or rocks along the edges, or they can run aground in shallow areas of the sea floor, generating friction to slow their movement like a spatula can hold back pancake batter. This buttressing of the ice shelves slows the spread of the ice behind them that sits wholly on land, keeping the ice sheet bigger and the ocean smaller. It is this land-based ice that raises alarms about sea level: If it reaches the sea faster, it is a new addition to the world’s oceans, so waters rise.
How does climate change affect this picture?
Ice shelves melt on their underside due to warmer water temperatures. During summer, warmer air temperatures can melt the ice from above. This causes thinning. As ice shelves thin, they can generate less friction along their sides or against shallow areas of the sea floor. And, beyond some threshold, ice shelves can completely break up, reducing buttressing to zero.
Oversimplifying a little, whether or not this calving becomes rapid will control whether or not the Antarctic ice sheet becomes an important contributor to sea-level rise by 2100 or before and whether it grows to dominate sea-level rise within the following century or so.
What we want to know is: What controls when ice shelves break off? When do things break fast, and when do they break slow? When does an ice shelf get lost versus merely getting shorter? The Ross Ice Shelf in Antarctica is in more or less the same position as when it was discovered in 1841 and for many millennia preceding. The Larsen B ice shelf sat there for 10,000 years and didn’t change a lot; then it went away in five weeks.
How are you trying to understand this?
Members of our broader research group are working extensively in the field. This especially involves the International Thwaites Glacier Collaboration, the major project to learn what is going on in the most vulnerable part of the most vulnerable ice sheet in the Antarctic, the West Antarctic Ice Sheet, which includes the Thwaites Glacier. After seasons are lost to COVID-19, a major expedition will be traversing down Thwaites, using radars, seismic sensors, and more to characterize the ice and its bed.
Other groups are working farther downstream, extending work that has been done on the ice shelf and in the ocean beyond. Thwaites is vast, larger than the state of Florida. It is some 80 miles across, making it arguably the widest glacier on Earth. Since the 1990s, scientists have reported on the increased velocity of its movement and the doubling of its contribution to sea-level rise. Its collapse would trigger meters of sea-level rise in the decades and centuries to come, hence its popular nickname in the media, Doomsday Glacier.
Our group, led by my colleague Sridhar Anandakrishnan, has also been working to understand the mechanisms of iceberg calving as part of a collaboration examining the great ice cliff of Helheim Glacier in southeastern Greenland. This work is supported by remote sensing, modeling, theory, and even laboratory experiments.
Really, seeing the great ice in the field is a transformative experience for many people. I had the remarkable experience of going to Greenland with 10 US senators as a guide to the ice, and they were truly impressed by the power and the potential for the loss of ice. We flew along the front of Jakobshavn Glacier, which drains the ice sheet into the ocean, watching a cliff taller than a 30-story building and knowing that about nine times that much extended below the water. It did not break while we were there, but when it did, seismologists around the Earth would see the earthquake waves and know what happened.
Do you and your team have any indication about what determines whether an ice shelf will break?
Much recent work, including papers led by Karen Alley (my daughter and a professor at the University of Manitoba) and by Shujie Wang at Penn State, offers an increasingly clear picture of how ice shelves break. How, where, and when ice shelves break are determined by multiple factors. One fascinating process goes something like this: Melting beneath ice shelves usually involves relatively warm, salty ocean waters flowing in and reaching the deep part of the ice shelf where it first starts to float. When the ice melts there, this freshens the ocean water a little, so it rises along the base of the ice shelf, generating turbulence that mixes in more warm water and drives more melting.
What we are learning is that meltwaters on the underside of an ice shelf don’t stay spread out across the whole base of the shelf. Instead, they localize into plumes that carve channels in the ice. These channels often form at the edges of the ice shelf. The channels at the edges cut upward, weakening the edges until they may break, removing the friction from the sides and transferring stress to where the ice starts to float. If that stress becomes enough, the whole ice shelf breaks off.
There’s a lot of fascinating information coming out of the Thwaites Glacier Collaboration. Is the news good or bad?
There surely is some bad news, but the work is also revealing some good news if you’re hoping that the sea level does not rise rapidly. There are ways that the glacier tends to stabilize where it is rather than retreating. For example, the rocks beneath are very warm and soft so that as the ice thins and removes weight, the rocks “bob up,” partially offsetting the ice loss and keeping the glacier grounded, aided by the accumulation of sediment. The bad-news discoveries include rapid thinning of the ice shelf from warm waters circulating beneath.
What are the challenges in predicting how much warming will ultimately cause the Thwaites Glacier to break apart?
Some of this is really difficult, especially where fractures are involved. Think about ceramic coffee cups dropped on hard floors. Sometimes the coffee cup just bounces, or the rim chips or the handle breaks off, but sometimes the whole thing shatters. Scientists can accurately predict the average behavior of a lot of coffee cups dropped on a lot of floors if you tell us the height of the drop, the type of floor, the type of cup, and a few other things. But predicting the exact behavior of the next cup dropped is really difficult, in part because the behavior depends on whether there are small cracks buried deep in the material of the cup, among others. Predicting exactly how much warming is needed to break parts of Thwaites will be harder than predicting coffee cups.
Sounds like there’s still a lot of uncertainty here. How should policymakers cope with that?
First of all, the uncertainties are not our friends. There is basically no way that sea-level rise can be notably smaller than expected. When we make the climate warmer, the ocean warms up. That makes the water expand, which raises the sea level. That’s relatively easy.
The glaciers in the mountains are doing what we projected decades ago: They really are melting. That takes water that was ice out of the mountains and puts it into the ocean, and that raises the sea level. Those are fairly easy predictions. There are no large uncertainties in those. The uncertainties are: Will the ice shelves break off? Will the flow of the big ice sheets change a lot, with the potential to drive these very large, rapid sea-level rises? So, the uncertainties are on the bad side.
In other areas of our lives, we tend to invest a lot to avoid the possibility of a catastrophe, even if we are not sure it is going to happen. The example I like to use is highway safety. We have highway engineers, we have crumple zones in the car, we have airbags and antilock brakes and seatbelts, and we have police out there trying to stop drunken drivers. We are not very likely to get killed by a drunken driver, but the catastrophe would be so bad if it happened that we invest a lot in heading that off.
What would make sense may be to think about sea-level rise and our response to it with the same sort of lens: There are things we can do to better understand why it happens and what the causes are. The next steps might be communities taking steps that reflect scientific findings, which, of course, have economic as well as social benefits.
You are also trained as an engineer and have thoughts about seawalls and their shortfalls. Do you see other paths forward that communities and cities working to defend themselves against rising seas might forge?
If calving really accelerates, it would be really, really expensive to design coastal defenses to protect against the larger possible rise in sea levels. How many billion dollars are you going to spend on defending against a meter of sea-level rise, and then you get 2 meters and get flooded? That’s partly why this uncertainty is so concerning.
Instead, we can get serious about research on the risk of rising sea levels. The cost of the research to reduce the uncertainties is small in comparison. This can seem self-serving because, while I’m an old guy and won’t do much of the research, it will be done by our students, colleagues, and friends. But I think you will find across the board in our field that we believe more research is cost-effective. Because of the chance of something worse happening — what’s that going to cost on the coasts?
And yet, you say you’re optimistic?
The optimistic part is that we can solve climate change now in ways that help the economy. When I was in school, the only things solar was good for were satellites and calculators. It was nowhere close to being competitive. But now, it is cheaper to add renewables than anything else. Putting more investment into renewable energy is economically favorable as well as environmentally favorable. When I was young, we did not know we could do it, but now we know we can.
This article originally appeared in Knowable Magazine, an independent journalistic endeavor from Annual Reviews. Sign up for the newsletter.