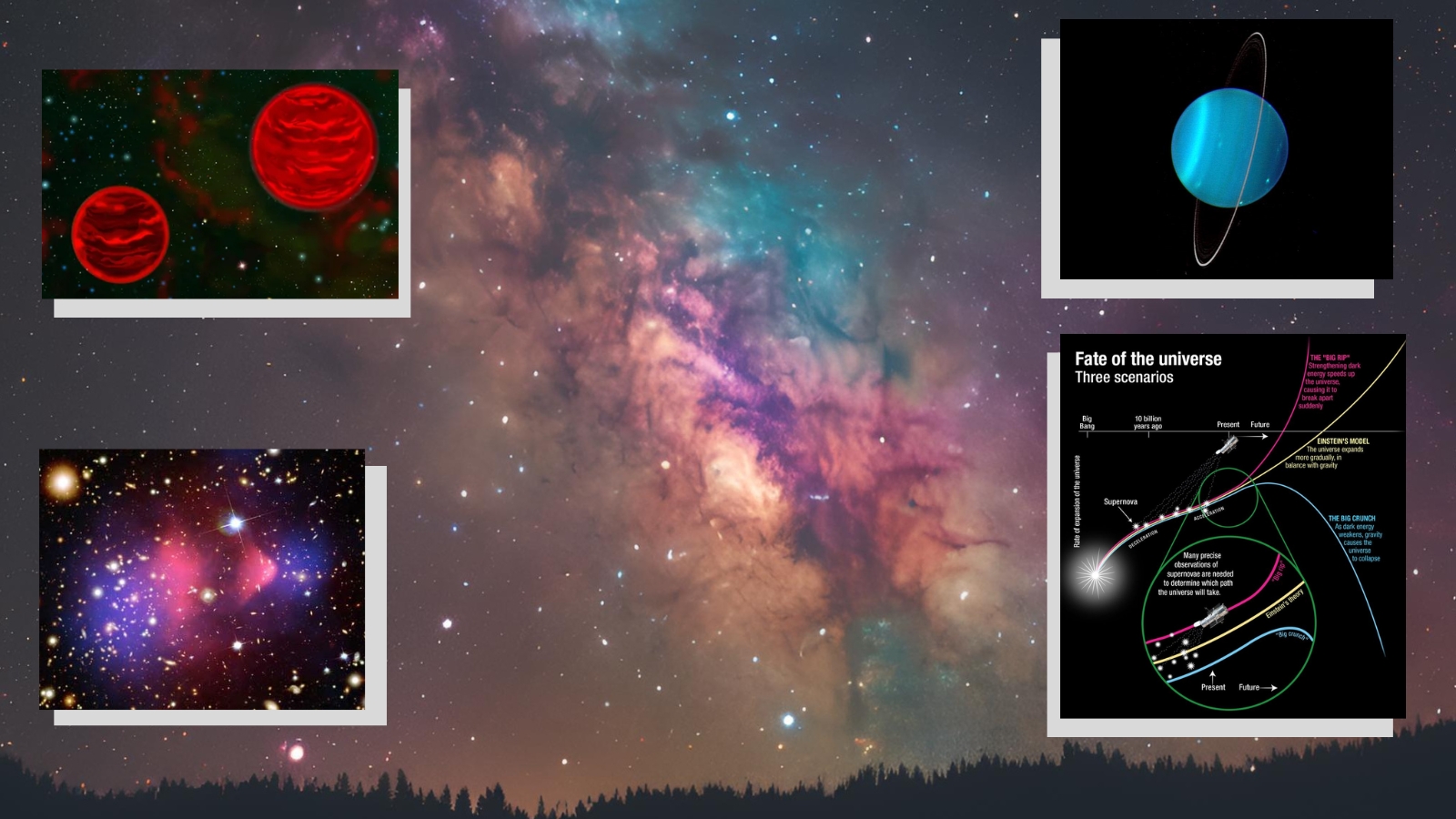
There's no doubt that humanity has made staggering leaps in understanding the cosmos since the beginning of the 20th century. However, there is still a great deal we don't know about the universe.
To put this into context, the "normal" matter around us that makes up stars, planets, moons, asteroids, animals, tables, chairs, computers and all other everyday objects accounts for only 5% of the matter and energy in the cosmos. That means we have no real understanding of what 95% of the cosmos — sometimes called the "dark universe" — actually is.
To compound this problem, even objects we do understand can throw us major curveballs. Consider the sun, the star we are most familiar with: We are still in the dark regarding the blistering heat of our star's outer atmosphere, the corona.
Here, Space.com explores some of the biggest space mysteries currently perplexing scientists.
When did dark energy "take over" the universe?
In the early 20th century, astronomer Edwin Hubble discovered that the universe is expanding, much to the shock of the physics community at the time, including Albert Einstein, who had believed the universe was static.
If this was a slap in the face to physics, what astronomers found at the end of the century was a kidney punch. While monitoring the distance to Type Ia supernovas, two separate teams found that not only is the universe expanding but that this expansion is also accelerating.
Dark energy was introduced as a placeholder name for whatever force is driving this expansion. We now know that dark energy, whatever it is, accounts for 68% of the universe's matter and energy budget. We'll come back to the other 27% of the universe's missing stuff in a moment.
However, the mystery we are highlighting here isn't "What is dark energy?" Sure, that is a considerable puzzle, but the arguably more pressing question is why dark energy started dominating the universe when it did.
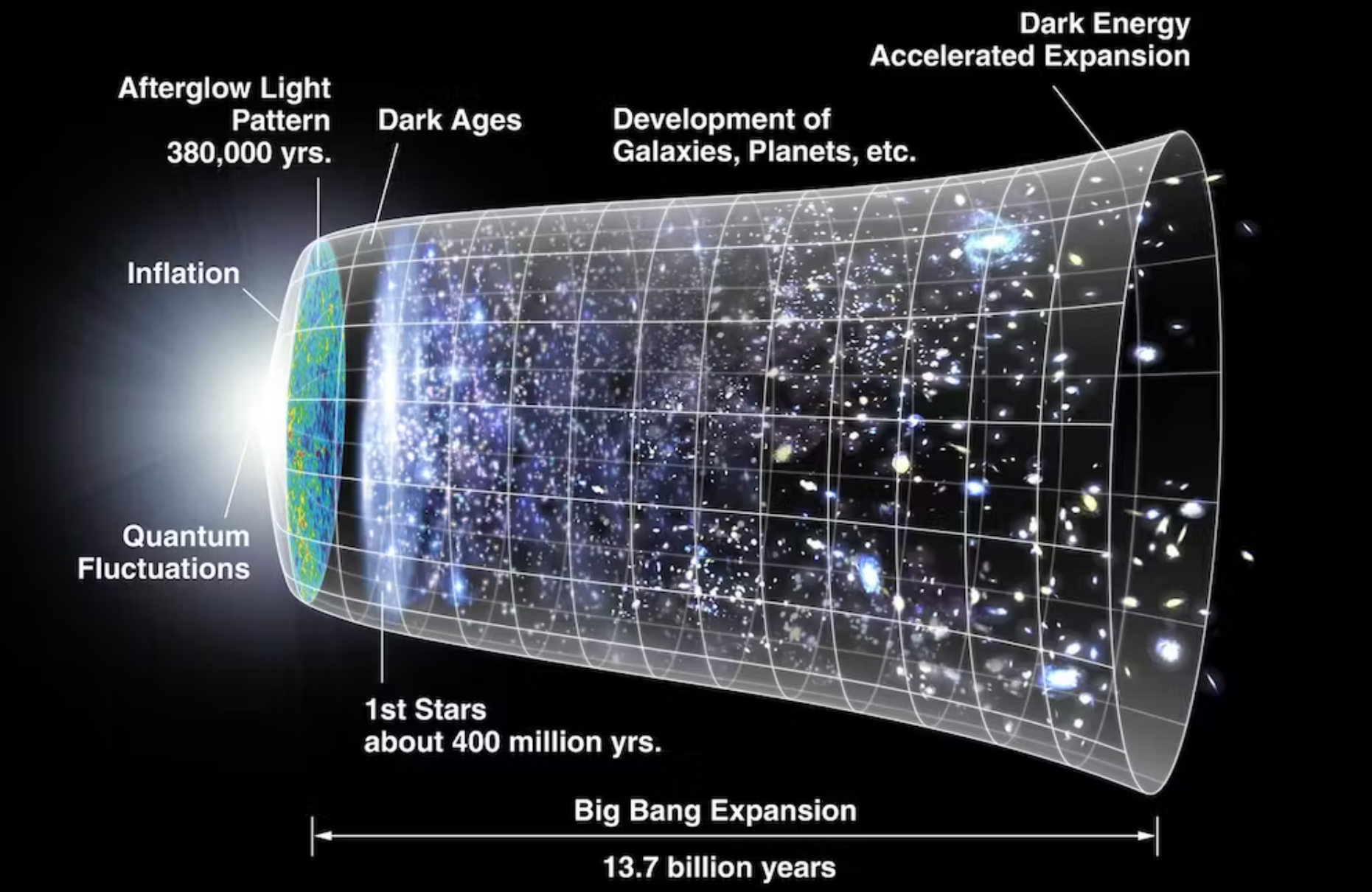
Think of the problem like this: At the beginning of time, the universe underwent sudden and rapid inflation, which we call the Big Bang. During this time, radiation dominated the universe. Around 47 million years later, matter began to form, and around 400 million years after that, the first galaxies formed and the expansion of the universe had slowed. Matter dominated this epoch. However, around 9 billion to 10 billion years after the Big Bang, something — dark energy — caused the universe to start expanding again. This expansion continues today, and it is getting faster and faster. We are in the dark-energy-dominated epoch of the universe. The question is, where was dark energy in the matter-dominated epoch, and how did it suddenly take over the cosmos?
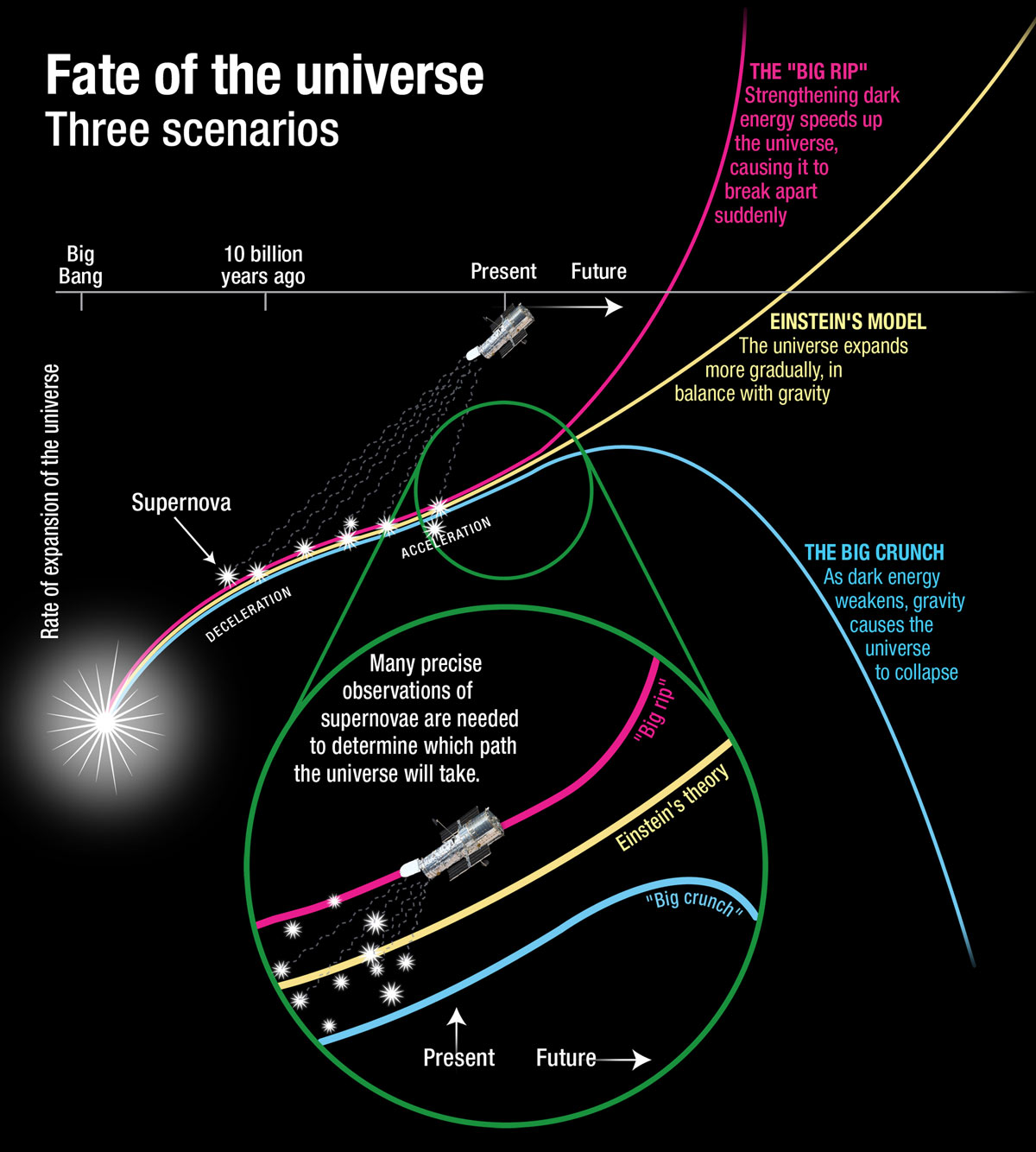
Answering these questions is the key not just to understanding what dark energy is but also to determining the final fate of the universe. Will the very fabric of space rip apart as a result of the continued acceleration? Or will matter again become dominant, causing the universe to "snap back" on itself like a stretched rubber band? The answer may lie in the history of dark energy, if we can decode it.
Read more: Dark energy could be getting weaker, suggesting the universe will end in a 'Big Crunch'
Why is the solar corona so hot?
One of the most curious small-stakes "local" space mysteries is the "coronal heating problem," which has puzzled researchers for decades. Scientists are still unsure why the diffuse cloud of plasma that makes up the sun's outer atmosphere, "the corona," is much hotter than the star's surface, the photosphere. This occurs even though the corona is effectively invisible because light from the photosphere completely overwhelms light from the corona. The corona can be seen clearly only during a total solar eclipse, when the moon covers the photosphere, blocking its light, or with an instrument called a coronascope, which does the same job artificially.
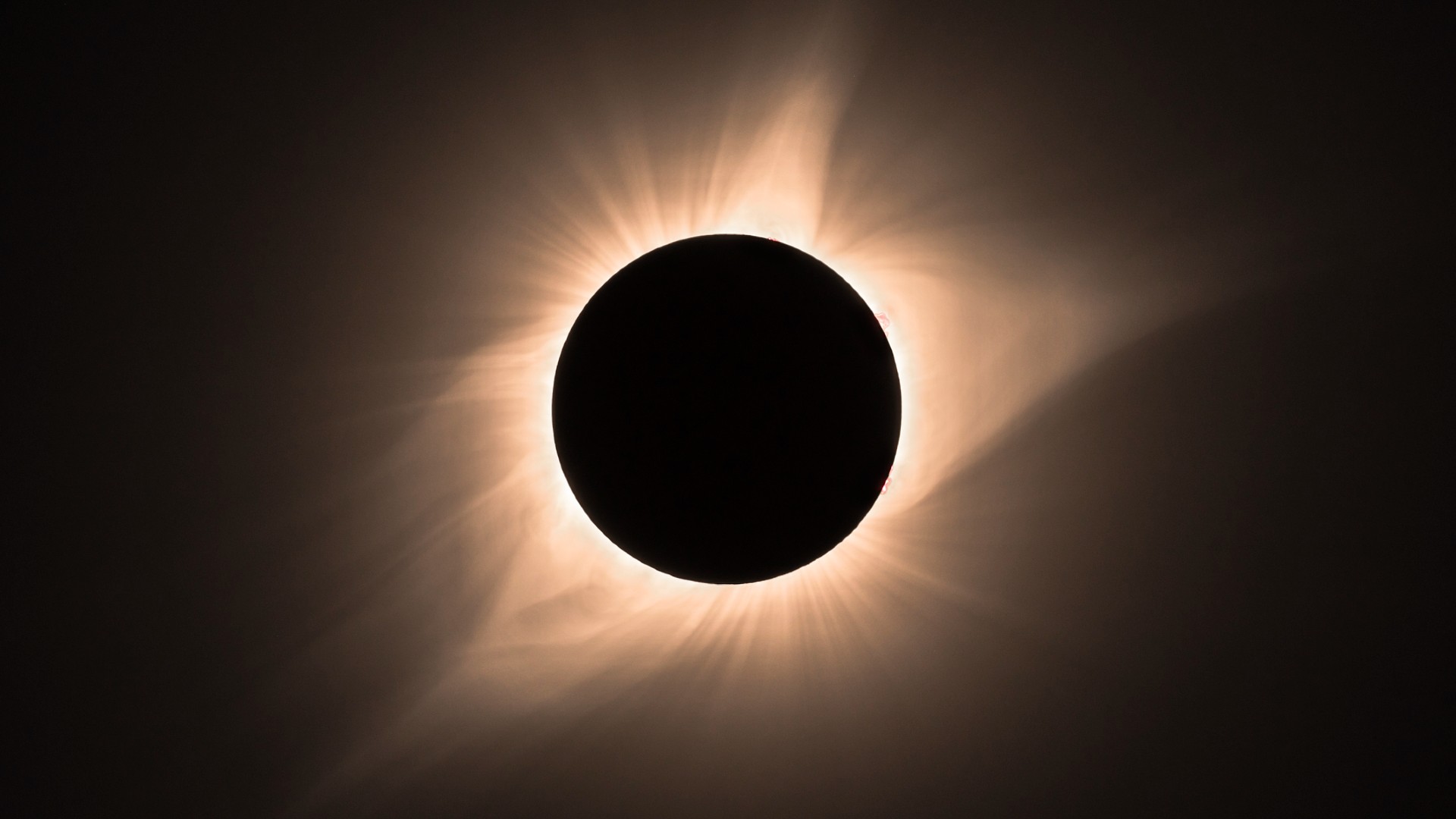
The difference in temperature between the two regions of the sun is significant: The corona can reach over 3.5 million degrees Fahrenheit (2 million degrees Celsius), while the sun's surface is a relatively balmy 10,000 F (6,000 C).
To understand why this is such a big mystery, consider that the vast majority of the sun's energy is generated at its core. That means the sun should get hotter the closer to the center you go — and all the regions of the sun obey this rule, except the corona. It would be like finding that your s'mores roast more quickly the farther from the campfire you held them.
Read more: Magnetic fields on the sun could solve long-standing solar heating mystery
How did supermassive black holes get so big, so fast?
While the James Webb Space Telescope (JWST) has answered many questions about the cosmos, there is one problem it has made significantly worse. The $10 billion space telescope has excelled in discovering supermassive black holes at the dawn of the universe, and while that sounds like a good thing, it has been a little worrying for scientists. Supermassive black holes have masses millions or billions of times that of the sun and are thought to reside at the hearts of all large galaxies. No single star has the mass needed to collapse and create a supermassive black hole, so scientists know these behemoths aren't born like more diminutive stellar-mass black holes, which have masses around 10 to 100 times that of the sun.
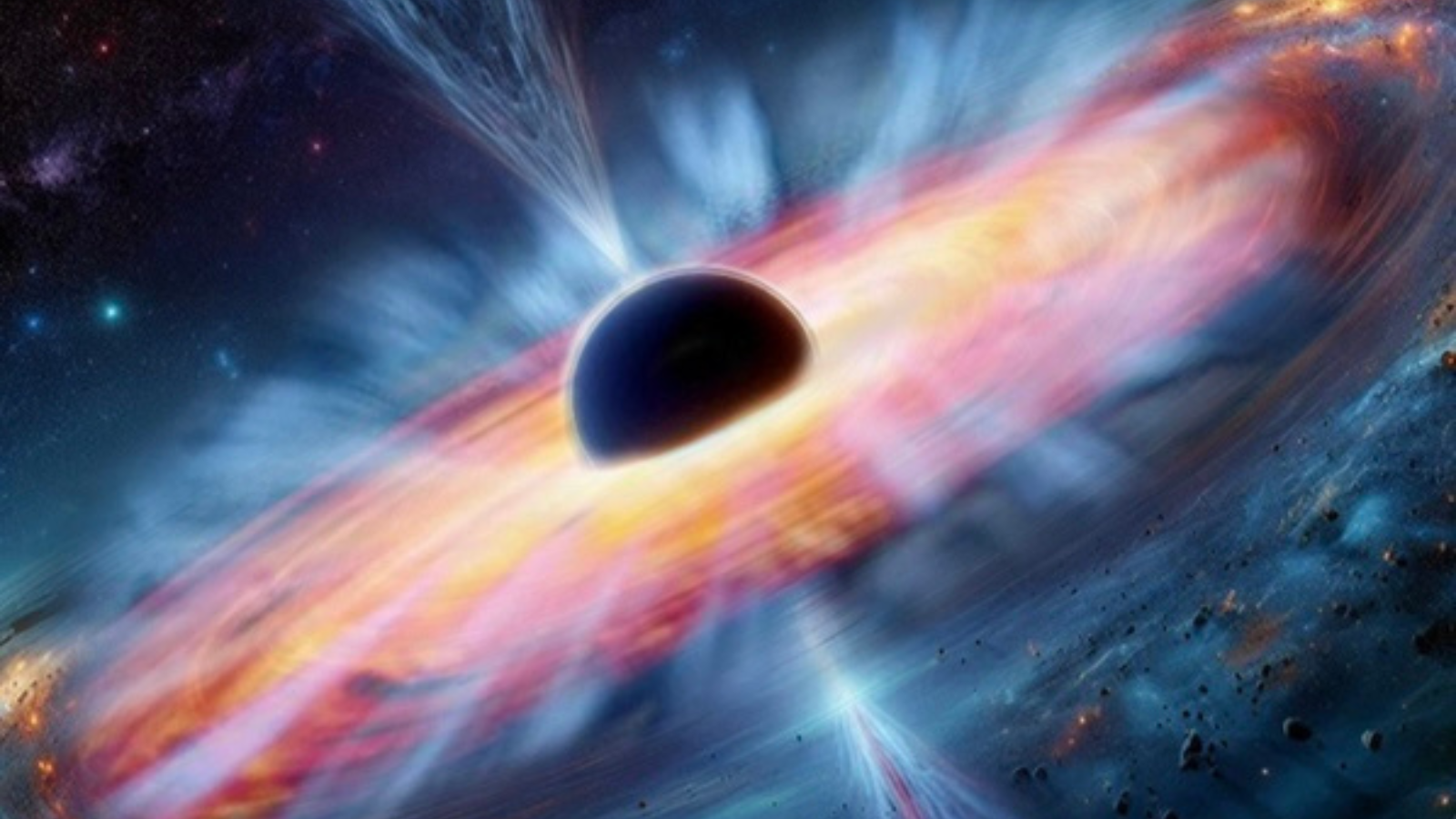
Scientists think supermassive black holes grow to monstrous sizes via merger chains of progressively massive black holes. Their growth is also thought to be aided by the rapid consumption, or accretion, of gas and dust from their host galaxies.
Here's the problem: As our understanding of that process currently stands, it should take at least 1 billion years. That means that, when JWST turns up supermassive black holes with millions of solar masses as early as 600 million years after the Big Bang, it's a real problem for our models of cosmic evolution. And these discoveries just keep coming.
"It's like seeing a family walking down the street, and they have two 6-foot teenagers, but they also have with them a 6-foot-tall toddler," John Reagan, Royal Society research fellow at Maynooth University, previously told Space.com. "That's a bit of a problem. How did the toddler get so tall? And it's the same for supermassive black holes in the universe. How did they get so massive, so quickly?"
Read more: James Webb Space Telescope catches monster black hole napping after 'overeating' in the early universe
What are JuMBOs?
All of the mysteries we've mentioned thus far have prestige and precedence because they've been troubling scientists for a decade or two. But a discovery made just last year shows there are lots of mysteries we haven't stumbled upon yet — "unknown unknowns," rather than "known unknowns."
This discovery came courtesy of JWSTin the form of "Jupiter-mass binary objects," or JuMBOs for short. Astronomers uncovered 40 pairs of JuMBOs unbound to any star in a star-birthing region of the Milky Way known as the Orion Nebula, around 1,350 light-years from Earth. JuMBOs are problematic because, as their discoverer, Mark McCaughrean, senior science advisor at the European Space Agency, pointed out, they aren't stars, and despite having planetary masses, they don't seem to be planets, either.
This curiosity is all about formation. The less massive a star is, the less likely it is to be binary. So, while around 75% of massive stars are found in binaries, this percentage drops to 50% for stars around the size of the sun and to 25% for the smallest stars. If JuMBOs with masses around that of Jupiter formed like stars, the number of binaries should be close to zero. That means finding 40 pairs in just one nebula should be impossible.
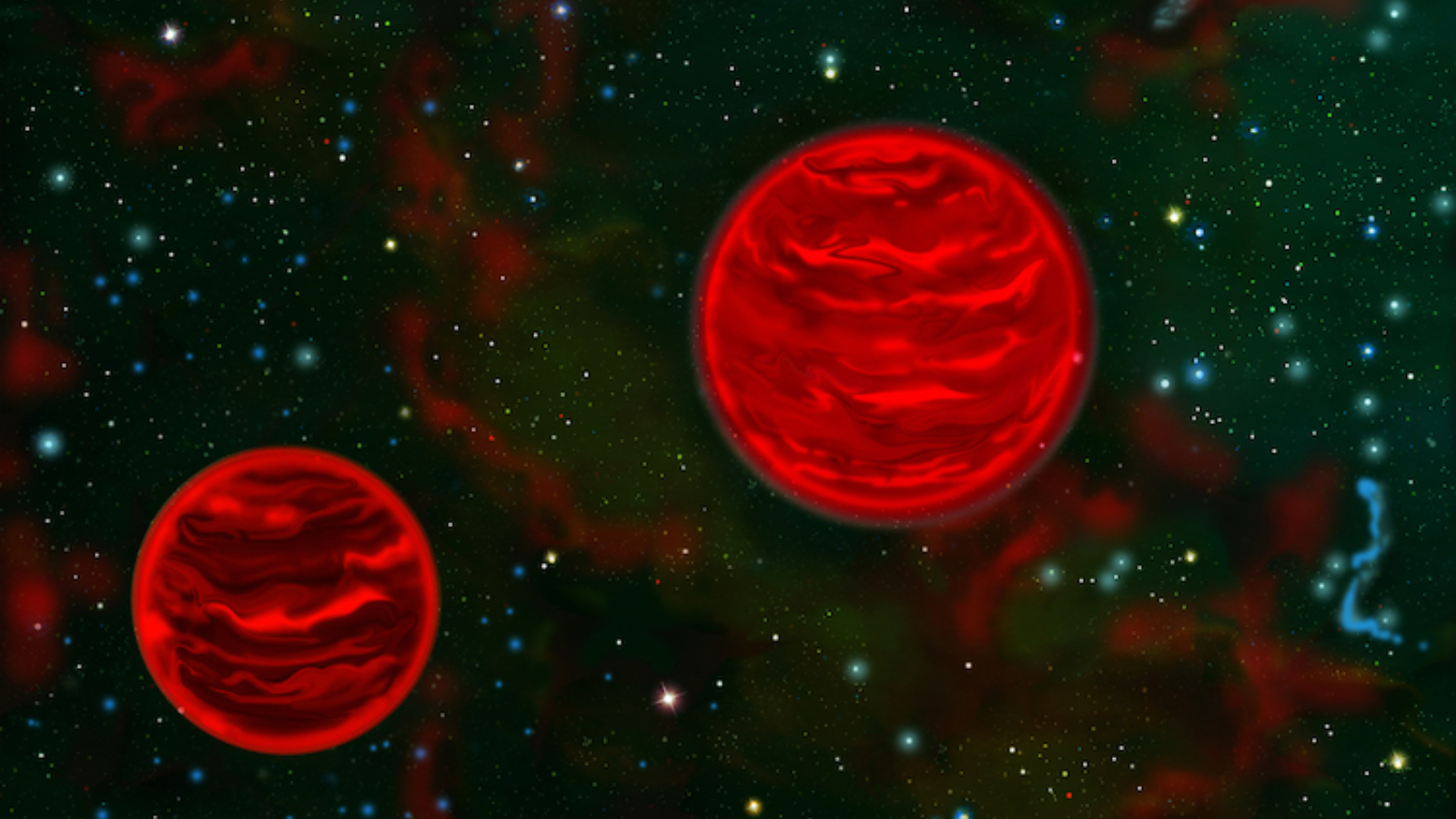
So why can't JuMBOs be born like planets? They can, but a problem with this emerges a little later. If JuMBOs formed like planets did, they would be born in rings of gas and dust around stars. These JuMBO pairings don't currently have a parent star, but that isn't too difficult to explain.
Planets can be ejected from stars to wander their galaxies alone as rogue worlds due to gravitational interactions with passing stars, or even with other planets in their system. The problem is, these ejection processes require a lot of energy and are quite violent. That means these ejection processes should be energetic enough to split JuMBO pairings. Of course, there could be some rare event that causes two planets to be ejected together without being separated. But again, the existence of 40 pairs of JuMBOs in Orion suggests that something more is at play here.
Scientists are now scrambling to explain how these curious objects formed.
Read more: The mystery of how strange cosmic objects called 'JuMBOs' went rogue
What lies at the heart of a black hole?
Black holes have made several appearances on this list, and it isn't just because we are obsessed with these cosmic titans (maybe a bit). Black holes are mysterious by design. A black hole's outer boundary is marked by its event horizon, the point at which nothing, not even light, can escape its grasp.
Nothing with mass can travel faster than light, which means anything passing the event horizon is on a one-way journey to the heart of the black hole. That means we can never hope to receive a signal from within a black hole. They are, and will forever be, shrouded in mystery.
Of course, where observation fails, we have theory, right? Wrong. Humanity's current best recipe for gravity, upon which our models of the universe are built, is general relativity, provided by Einstein in 1915.
Less than a year after this, in December 1915, Black holes first alerted themselves to scientists theoretically due to the fact that solutions to the equations of general relativity produced by German astronomer Karl Schwarzschild (as he served on the frontline during World War I) produced calculations indicating there could be an infinitesimally small point in space at which a huge amount of matter could condense to cause the equations of general relativity to go to infinity.
Scientists call this point a "singularity," and it is very troubling because it means that, at the heart of a black hole, all the laws and theories of physics collapse like the dying stars that give rise to black holes in the first place.
Read more: Was Oppenheimer, the father of the atomic bomb, also the father of black holes?
What is the secret of dark matter?
Remember that missing 27% of the universe's matter and energy budget? The other element of the universe is dark matter, and scientists have little idea what it is. However, we do have a few more clues than we do for dark energy. For example, we are fairly confident about what dark matter isn't.
Dark matter doesn't interact with photons (particles of light), or when it does, the interaction is so weak that we can't see it. Ditto for particles of "everyday" matter, which is composed of atoms comprising electrons, protons and neutrons. Thus, we know that dark matter isn't composed of "normal" matter.
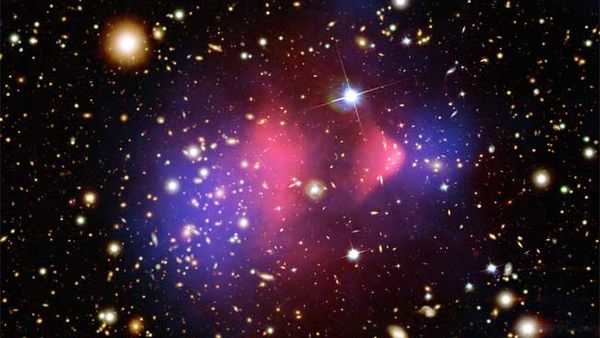
The only way we can infer the presence of dark matter is via its interactions with gravity. This influences and shapes the fabric of space and everyday matter, and light that we can see reacts to this. For example, dark matter was discovered when American astronomers Vera Rubin and Kent Ford discovered that some galaxies are rotating so fast that the gravitational influence of their visible matter would not be sufficient to hold them together. The gravity of dark matter is the glue that prevents the galaxies from flying apart.
The fact that dark matter can't be composed of normal baryonic matter has spurred the search for a particle that could account for this mysterious substance. The leading suspect thus far is an incredibly lightweight particle called the axion. Yet, despite intense interest, a candidate for dark matter has defied discovery.
This continued particle detection failure has led some scientists to revisit the idea that tiny primordial black holes left over from the Big Bang might account for dark matter. But these cosmic candidates, like axions, remain purely hypothetical.
Read more: Happy Dark Matter Day! Meet the usual — and unusual — suspects in this cosmic mystery
Why the constant problems?
When Einstein's theory of gravity, general relativity, was used to describe the universe, it produced a cosmos that wouldn't "sit still." That was a problem for Einstein because, although he was never afraid to challenge a paradigm, he agreed with the consensus at the time that the universe was static.
To combat this, Einstein introduced a "fudge factor" to his equations called "the cosmological constant” represented by the Greek letter lambda, that would counteract gravity and stop the universe from contracting. In 1929, when Hubble convinced Einstein that the universe was expanding, the physicist consigned the cosmological constant to the theoretical trash can, allegedly describing the introduction of lambda as his "greatest blunder."
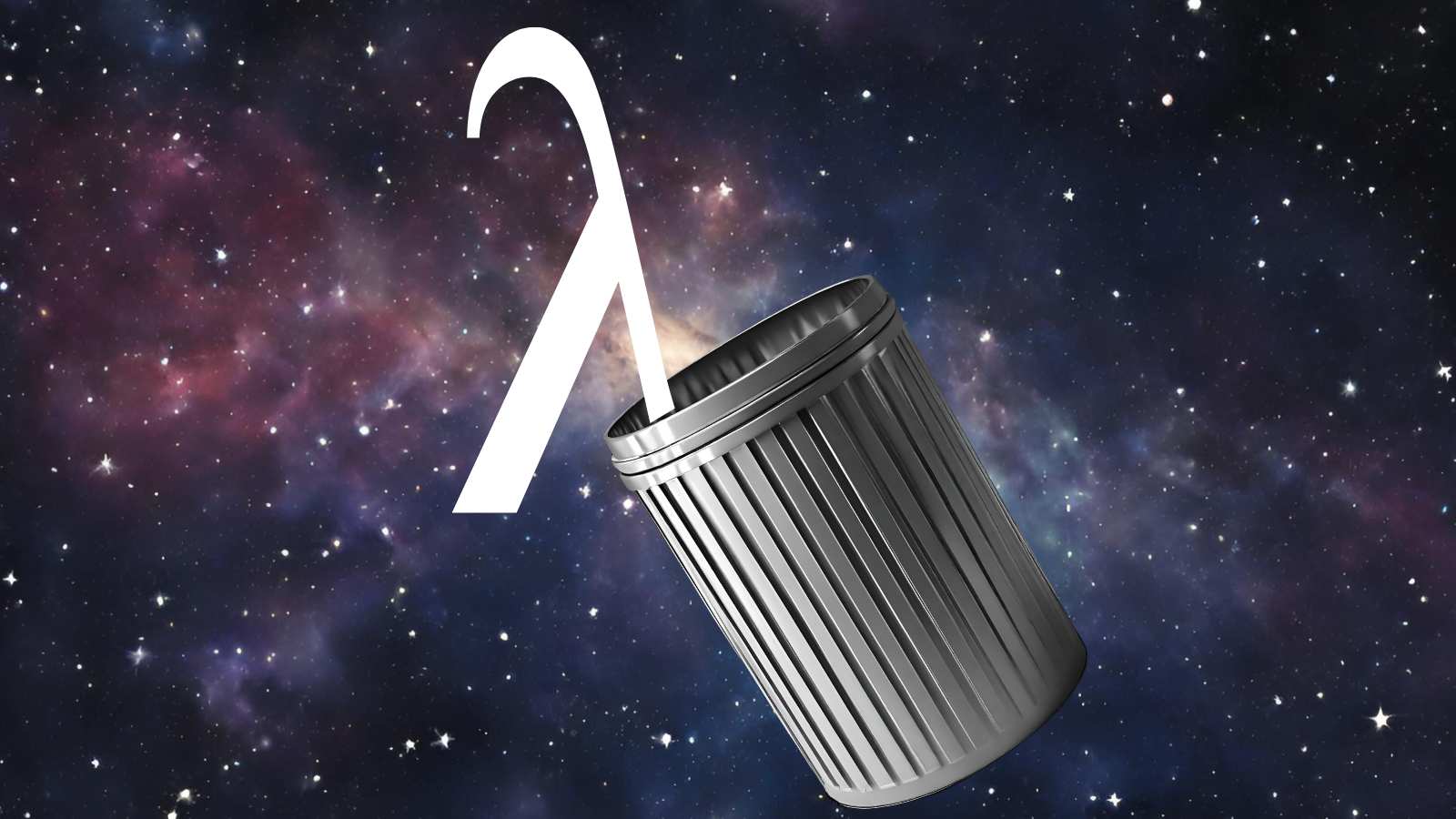
But the revelation of the accelerating expansion of the universe in the 1990s prompted the return of the cosmological constant, which is still represented by lambda but in a new role: It describes the action of dark energy in stretching the fabric of space faster and faster.
Even in its new form, however, lambda is still a major headache for scientists. That's because the values for lambda delivered by observations of distant supernovas differ from the value predicted by theories of quantum physics by as much as 10 to the power of 121 (1 followed by 121 zeroes).
The cosmological constant has been described as the "worst prediction in the history of physics," and there seems to be no resolution to this vast disparity on the horizon.
Read more: In a way, Space.com and the dark universe grew up together
What smacked Uranus?
OK, enough Uranus jokes. We're serious about this: Uranus is a unique and strange solar system planet because, unlike all the other worlds in our corner of space, it rolls around the sun on its side. The icy planet is tilted at over 90 degrees in relation to the plane of the sun, as are the orbits of its 28 moons and its faint rings of icy material. All of the other solar system planets have orbital tilts of less than 30 degrees.
Additionally, Uranus stands out because it rotates on its axis very quickly; a day on the planet lasts just 17 hours.
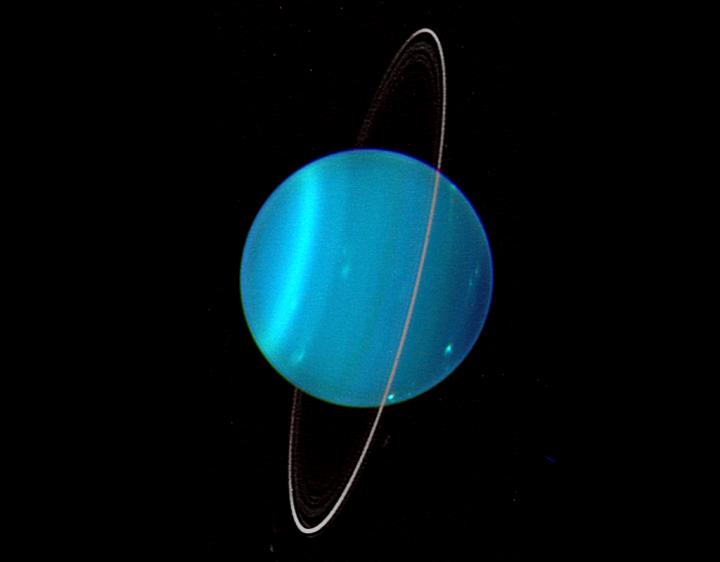
Scientists think these unusual characteristics resulted from an object one to three times as massive as Earth crashing into Uranus billions of years ago as the solar system was forming. But when researchers run simulations of this collision, the impact of a body massive enough to drastically shift the solar system's third-largest planet produces debris that is too massive given the size of Uranus' moons.
Scientists hope to solve the mystery of this ice giant's strange qualities with future missions.
Read more: The yearning for Uranus: A far-out world with a tale to tell